57 min read • Healthcare & Life Sciences
The brave new world of synthetic biology
Major impacts, significant challenges
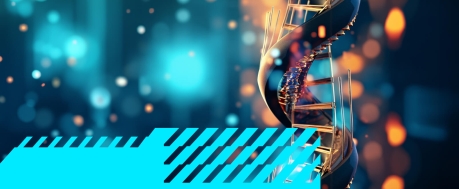
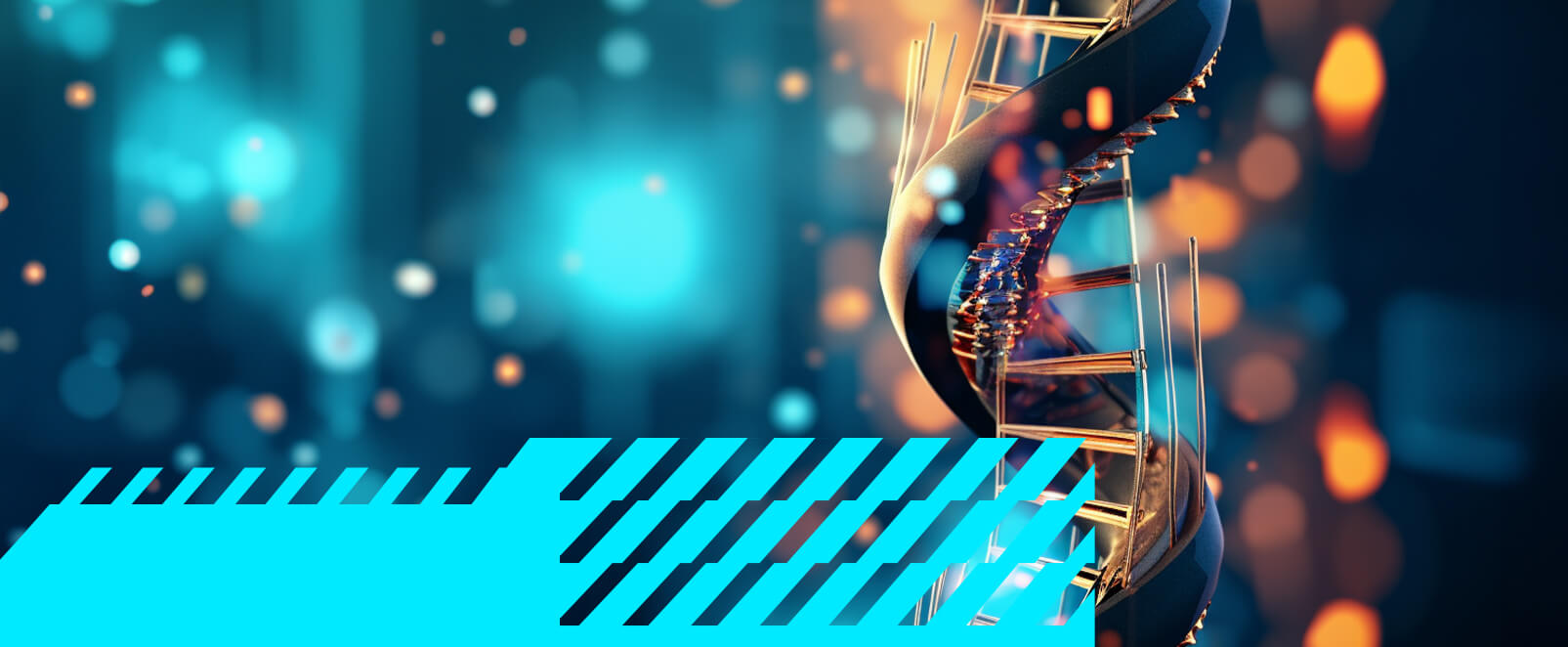
Executive Summary
“What I cannot create, I do not understand.”
— Richard Feynman, theoretical physicist
While the 20th century was in many ways the century of the industrialization of chemistry, the 21st century is promising a similar revolution in biology. The revolution’s foundation began with the discovery of DNA in the 1950s and expanded to core technologies that help to understand, analyze, and manipulate our genetic codes as well as the proteins and, ultimately, the cells and organs that underlay human biology and extend to the universe surrounding us. These findings have led to numerous groundbreaking discoveries and the expanding potential to understand, detect, and treat diseases. We are also increasingly seeing biological systems used outside of the life sciences in a wide range of fields.
In recent years, the discussion increasingly has centered on synthetic biology, or SynBio, a term coined in the 1980s when scientist Barbara Hobom used it to describe bacteria that had been genetically engineered using recombinant DNA technology. The term has evolved significantly since then to be used more broadly and sometimes controversially.
While SynBio has already increased our understanding of biological systems and led to the availability of new tools for their manipulation and diagnostics, more recent advances in computing power and development of tools such as artificial intelligence (AI) and machine learning (ML) are leading to a further rapid acceleration in innovation. The impacts have the potential to be disruptive across many industry sectors — not just healthcare and life sciences and food and agriculture, but also industrial, chemicals, manufacturing, consumer goods, energy, and IT.
With this increasing impact across multiple disciplines and ecosystems comes an expanding need for communication and critical discourse around the implications of SynBio for society. While SynBio offers the potential to generate foods like milk or meat without animals and their associated environmental impact, we require better regulation to control quality and safety and need to understand the global and economic implications in this field. Recent examples such as the HeLa cell case and the debates around golden rice or what we are allowed to call “milk” show that we need to better address some basic questions: Who owns a gene or a cell? What level and speed of change are we willing to accept as a society? What are the risks of these new technologies? While gene and cell therapy offer the opportunity to cure serious and debilitating diseases such as muscular dystrophy or hemophilia, innovations are already enabling, for example, the synthetic creation of human embryos. What implications do these have for our future development?
Based on extensive Arthur D. Little research supported by surveys and interviews with 28 global experts in SynBio technology and applications, this Report aims to provide a comprehensive overview of the current status and prospects for SynBio, including what it comprises, the present state of technological developments, the existing development market and key player landscape, current and prospective applications, and how businesses should prepare for the future. Overall, we conclude that:
-
SynBio is an extremely diverse field. SynBio is unique in the breadth of its potential applications — from healthcare to food, manufacturing, industrial processing, and even the digital industries. The maturity of its technologies range from the embryonic to the fully commercialized. In considering its prospects, it is important to take a sufficiently granular approach.
-
SynBio technologies could have massive transformational impact in a 20-year time frame. Technologies like predictive genetic engineering, DNA synthesis for complex organisms, DNA-based storage, and AI-powered applications could transform our industries, our ability to engineer living systems, and how we cope with climate change.
-
Many SynBio applications are poised for growth. Forecast growth rates for SynBio are >20% CAGR to 2030 overall, especially in healthcare and life sciences and food and agriculture. Drivers for growth are strong, both in terms of technology push (increasing investment, lower technology costs, growing ecosystems) and market pull (especially sustainability, healthcare needs, and manufacturing efficiency). The current market is predicted to grow from around US $15 billion in 2023 to $70 billion by 2030. Perhaps more importantly, if currently developing SynBio technologies mature successfully, SynBio could have a huge impact, disrupting sectors comprising as much as 15%-20% of the entire global output in several areas in the coming decades, including:
-
Healthcare and life sciences. A large range of application areas enable personalized and potentially curative treatments as well as improvements in diagnostics and drug design and development. In the future, we expect more sophisticated treatments, greater protection from more diseases, and new applications for degenerative and genetic conditions.
-
Food and agriculture. Applications are extensive, ranging from crop domestication to synthetic food and bioremediation, with further developments in all these areas expected in the coming years.
-
Industrial and consumer goods. Applications include novel/specialty products, raw material replacements, sustainability, and process performance improvement.
-
Energy. Applications include biofuels, bioelectricity, bio-batteries, remediation, and process improvement for traditional fuels.
-
IT and tech sector. DNA-based data storage is the most promising research area but is still only at the proof-of-concept (PoC) stage.
-
-
Challenges and barriers remain high with several critical uncertainties. In many SynBio applications, development has been ongoing for decades, often driven more by technology push than market pull. Critical uncertainties include skill/competency gaps, achieving standardization of toolsets and systematization of the knowledge base, adverse public perception, ethical/biosecurity issues, availability of funding and investment, difficulties in scaling up to production levels, and poor economics compared to conventional technologies, which could hinder market growth.
-
Companies should ensure a suitable SynBio strategy is in place. Given strong drivers, accelerating technology developments, and potentially huge impacts, companies should be prepared for exponential growth that could result from breakthroughs. Given its diversity, companies need to consider carefully which fields are relevant for their business and conduct realistic market-based assessments. Key steps include:
-
Identify potential opportunities. Pinpoint those areas of the business that could be disrupted, or new opportunities created, by current or envisaged future SynBio technologies. Consider how SynBio could enhance current performance, enable new products or processes, enhance sustainability, and/or improve efficiency or customization.
-
Assess attractiveness and develop strategy. Take a market-led approach to assessing potential business cases and develop a scenario-based strategy, considering the barriers to be overcome and the critical uncertainties that remain.
-
Develop capabilities. Understand where and how to bring together the required capabilities within the ecosystem. This is especially important given SynBio’s multidisciplinary nature and shortages of specialist skills.
-
Maintain ecosystem presence. It is vital to engage in regulatory and ethical discourse and respond rapidly if and when applications become exponential.
Preamble
I am a fan of technology. Yet at the same time, I am extremely concerned about its negative externalities. Negative externalities on our children, on society, on our institutions, or on our businesses.
This concern is not new. In fact, I particularly remember reading Brave New World by Aldous Huxley in high school as a teenager. In this novel, Huxley presented a seemingly wonderful world where a mix of totalitarianism and technology resulted in an extremely stable global society without war or conflicts. By all appearances, it was the best of all possible worlds. However, individual freedoms and free will almost completely disappeared. That was my introduction to technological ambivalence.
While preparing this Report on synthetic biology with the Blue Shift team, Huxley’s Brave New World came back to me like a genetically modified “madeleine de Proust,” and I could not help but think about all the dystopian scenarios. Why? Because SynBio encompasses a multitude of potential threats and negative impacts that could arise from its misuse or unintended consequences: deadly pathogens, gene drive (which can be used to propagate a particular suite of genes throughout a population) technology, environmental and health risks, or the DIY biology movement (a movement of citizen scientists interested in SynBio experiments, which raises concerns about the misuse of easily accessible and low-cost technologies like CRISPR [defined as clustered regularly interspaced short palindromic repeat] by individuals with little prior knowledge of the field). And the list goes on!
But at the same time, I am totally amazed by the fact that most any industrial sector could leverage SynBio — not just life sciences. In this Report, we focus primarily on the positives SynBio will bring to business, society, and humans.
Before diving into it, I would like to share with you another very intriguing anagram:
“Synthetic biology”
transforms into
“hit by Scientology”
As always, anagrams move in mysterious ways.
– Albert Meige, PhD
1
What is synthetic biology & why is it important?
Mankind has long questioned the nature of life, endlessly exploring the topic through religion, philosophy, art, and science. Since the mid-20th century and the discovery of DNA, the field of SynBio has provided insights into some of the “big questions” (see Figure 1).
For example, SynBio has shown that we can synthetically create new and different functions in living organisms and harness living cells to perform nonbiological tasks. In 2023, researchers at the Weizmann Institute of Science, Israel, claimed to have grown an entity that closely resembles a 14-day human embryo, without using sperm, eggs, or a womb.[1]
Defining SynBio
Today, definitions of SynBio vary but most center on the basic concept of engineering biological systems for useful purposes. Examples include:
-
“The design and construction of new biological parts, devices, and systems, and the redesign of existing, natural biological systems for useful purposes” (Nature)
-
“A field of science that involves redesigning organisms for useful purposes by engineering them to have new abilities” (US National Human Genome Research Institute)
-
“The use of modern technologies and computation to engineer biology to solve problems related to the environment and human health” (University of California San Diego [UCSD] Synthetic Biology Institute, USA)
-
“A multidisciplinary field of science that focuses on living systems and organisms, and it applies engineering principles to develop new biological parts, devices, and systems or to redesign existing systems found in nature” (Wikipedia)
A 2023 Blue Shift global expert survey[2] carried out as part of this Report explored what distinguishes SynBio from related fields. It found that experts commonly identify convergence, engineering principles, and systems thinking as key features (see Figure 2).
While opinions broadly concur with these three aspects, experts do not fully agree on whether the source materials must be living organisms or if they can be created synthetically. For the purposes of this study and its ultimate focus on applications and impacts, we concluded that it is more useful to define the field in terms of what it produces and how it works, rather than the nature of its raw materials.
We therefore propose a practical definition of SynBio that embodies the following key features: “Engineering, modifying, or creating novel living or hybrid[3] biosystems for useful purposes.”
Supporting this definition, SynBio involves three features:
-
The convergence of multiple disciplines
-
Bridging life and physical sciences
-
At the boundary between naturally occurring and artificial worlds
-
Living or hybrid systems
-
Biomimetic engineering approaches can also be part of the SynBio toolbox, although mimicking biological systems is not a SynBio aim, per se
-
-
Applied engineering principles
-
Intentional engineering of living systems to achieve specific goals and functionalities
-
-
Systems thinking and modularity
-
Decomposition of biological systems into interchangeable modules
-
Understanding how individual parts behave and interact within the overall system
-
The spectrum of SynBio applications
SynBio covers a wide range of potential applications (see Figure 3), characterized by their degree of novelty, from more basic enhancement of existing capabilities to the complete creation of entirely new living systems.
At the next level of detail, it is useful to split the functionalities of SynBio into categories across three broad aims (see Figure 4).
As Figure 4 illustrates, SynBio can be used for making completely new products (biological and nonbiological), enhancing the performance of existing products, and improving production and manufacturing processes for the same products. In the latter category, improved sustainability is an important benefit, although better efficiency and precision are also key.
What drives advances in SynBio?
Although SynBio’s origins go back to the 1950s, breakthroughs have accelerated considerably over the last 20 years, as shown in the timeline in Figure 5.
Over the last decade, SynBio progress has further accelerated due to both technology push and market demand:
-
Technology push — convergence between ongoing developments in life sciences and physical sciences (see Figure 6), including more powerful and accurate gene-editing tools
-
Market demand — the need to meet growing demands for:
-
Greater sustainability (e.g., renewable raw materials/energy)
-
Improved healthcare (e.g., faster, lower-cost drug development; improved diagnostics; more targeted therapies)
-
Greater food security (e.g., improving yields at time of climate change and population growth)
-
Innovation (e.g., more tailored products, transformative advances in computing, and other radical new applications)
-
This combination is driving increasing public and private investment and partnerships across SynBio ecosystems. Examples within the US include:
- US government SynBio research funding increased to $161 million in 2022, with public funding totaling $820 million over the last five years.
-
The US private sector invested $8 billion in SynBio in 2022.
-
Institutions including Northwestern University, USA, and the Massachusetts Institute of Technology (MIT), USA, have received grant awards for SynBio research projects involving interdisciplinary teams.
-
New government-sponsored collaborations have launched, such as the SynBio partnership involving Ginkgo Bioworks, Geno, and Antheia.
-
New consortia have formed to develop cross-platform solutions. For example, Microsoft, Lenovo, and Twist Bioscience are collaborating to explore cross-platform work, such as through the potential of DNA data storage.
Increased activity is fueling predicted growth of the global SynBio market of 24% CAGR to over $70 billion in 2030, from around $15 billion in 2023 (see Figure 7). This covers just the provision of services, tools, know-how, and products to enable the application of SynBio by end users in markets such as healthcare, food, and industry. It does not include any potential incremental revenue these end users may realize using SynBio tools, the impacts of which we outline in the final part of this section and in Chapter 2.
Challenges to SynBio growth
Despite demonstrating impressive growth potential, SynBio development faces large-scale obstacles. These are wide ranging, from biosecurity/ethical issues to technical, economic, and consumer challenges.
Biosecurity & ethical issues
Altering biological organisms — or creating completely new ones — raises biosecurity challenges around potential unintended environmental consequences, or the deliberate misuse of SynBio by bad actors. It also leads to an ethical debate around what is deemed acceptable when it comes to changing or creating forms of life, and a blurring of the boundaries between “animate” organisms and “inanimate” machines, as detailed in Figure 8.
Global regulation is central to both ensuring biosecurity and providing reassurance around ethical concerns, such as by adopting and enforcing key principles and codes of conduct with researchers, companies, and countries.
However, regulations and guidelines are still evolving. Some overall principles have been defined, such as the long-established and internationally recognized Convention on Biological Diversity (CBD). This includes provisions related to SynBio, for example, around ensuring the safe handling, transfer, and use of living modified organisms (LMOs) produced using SynBio techniques.
However, these principles have yet to be consistently applied at a global regulatory level. Currently, fragmented national and regional regulatory frameworks equates to limited guidance over a coherent approach to the responsible use of SynBio.
Guidelines are also being developed around ethical issues. For example, codes of conduct, such as those set up for scientific research, have been created by organizations like the International Society for Systems Biology (ISSB) and the Synthetic Biology Leadership Council (SBLC). Bodies such as the World Health Organization (WHO) are forming similar guidelines.
However, along with the pace of emerging technologies, regulations are changing rapidly as well. This means that understanding and navigating different biosecurity and ethical issues remains a challenge for SynBio players.
Technical, economic & consumer challenges
At the same time, there remain significant technical, economic, and consumer acceptance barriers when it comes to accelerating the use of SynBio in many areas. These include the difficulties of scaling up processes from the lab to full production, poor cost-competitiveness with existing alternatives, a lack of sufficiently powerful technology, a need for large-scale investment, and potential consumer pushback at SynBio-derived products viewed as dangerous or threatening in some way (see Figure 9).
The transformative potential of SynBio
While it currently faces significant challenges, SynBio’s potential impact on end-user industries is vast. SynBio applications have the power to fundamentally change some of the largest industry sectors across the globe, helping solve critical challenges that threaten humanity’s future, such as those around sustainability, health, and food supply.
These industry sectors, including agriculture, healthcare, manufacturing, and data processing, represent about 15%-20% of the current global output, demonstrating SynBio’s potential significance if it can overcome the challenges and uncertainties outlined in the section above. Taking the example of the US, these disrupted sectors make up around 20% of current GDP, covering approximately $5 trillion, as illustrated in Figure 10.
Given this far-reaching and diverse potential, players in the sectors concerned cannot afford to ignore the ongoing progress of SynBio development — especially in view of the increasing strength of the drivers on both the technology and market sides.
2
The scientific & technical landscape
Although boundaries can blur, the development landscape for SynBio can broadly be split into three groups (see Figure 11):
-
Product developers — creating customer products and applications that leverage SynBio know-how and toolsets
-
Tool & technology developers — developing tools and technologies that enable SynBio products and applications, including techniques, hardware, software, and systems
-
Underlying academic research — creating basic and applied understanding of the science underpinning SynBio
As with all models, the distinctions are not always clear. For example, some tool and technology players, such as Asimov and Arzeda, are also extensively involved in product development, often in partnership with product-based businesses.
This section of the Report focuses on the academic research and tool/technology development part of the stack. We cover product developers and applications in more depth in Chapter 3.
Underlying academic research
Academic research in SynBio is extensive and covers a wide range of domains, reflecting its multidisciplinary nature. US and European institutions currently lead in investments in SynBio research, although investment in Asia is on the rise. Select examples of major academic players and their main areas of research include the following (illustrative only, non-exhaustive).
US players
MIT Department of Biological Engineering
Focusing on a wide range of relevant research for medical and life sciences applications, MIT’s centers include:
-
The Center for Multi-Cellular Engineered Living Systems — a multidisciplinary group studying purpose-driven living systems with multiple interacting living components
-
The Center for Biomedical Innovation — develops new approaches to biomedical technology, such as the structure and function of biomembrane proteins for applications in sensors
-
The Center for Neurobiological Engineering — focuses on new tools for experimental investigation of the nervous system and engineering neurons, neural tissue, and their interactions with cells, devices, and prosthetics
Northwestern University: Center for Synthetic Biology
The Center’s research is based around four themes:
-
Cell-free systems — biochemical engineering with cell-free biology activating basic cellular processes (e.g., translation) without live intact cells
-
Mammalian systems — developing novel platforms and therapeutic strategies based on custom-programming novel cellular functions
-
Enabling technologies — high-throughput experimentation tools that will allow the optimization and understanding of multi-component biosynthetic transformations, including work on engineering platforms, parallel expression of proteins, and computational analysis to identify design rules in the data
-
Ethics and societal impact — technology gaps in low- and middle-income countries, exploring the new bioethical questions that arise from SynBio development and developing ethical training for scientists
Stanford University: Synthetic Biology & Bioengineering
These two programs explore broad-based SynBio research, including protein engineering, microbiome studies, systems biology, bioinformatics, metabolic engineering, neuroengineering, biomechanics, medical devices, medical imaging, molecular engineering, microscopy, biomaterials, tissue engineering, omics/genomics, gene therapy, microfluidics, agrobiology and plant health, frugal science, and bio policy.
European players
Switzerland: ETH Zurich
The SynBio group focuses on developing and applying the tools and methods of biological computing and synthetic biology in the mammalian context, based on three pillars:
-
Fundamental, enabling technologies for precise manipulation and reprogramming of mammalian cells
-
Applying these tools to unmet needs in biotechnology and translational research
-
Development of gene and cell therapies powered by biological computing
UK: Imperial College Centre for Synthetic Biology
Research focuses on novel methods to accelerate the design-build-test-learn cycle as well as multi/interdisciplinary research, including:
-
Computational modeling and ML approaches
-
Automated platform development and genetic circuit engineering
-
Multi-cellular and multi-organismal interactions (including gene drive and genome engineering, metabolic engineering, in vitro/cell-free SynBio, engineered phages, and directed evolution)
-
Biomimetics, biomaterials, and biological engineering
The College also hosts SynbiCITE, the UK’s innovation and knowledge center, created to accelerate and promote the commercial exploitation of SynBio research and engineering biology applications. This includes the London Biofoundry, aimed at designing, engineering, and functional characterization of synthetic DNA.
Germany: Max Planck Research Network in Synthetic Biology
The network comprises 20 research groups from nine Max Planck Institutes. Research clusters include enabling technologies, life-mimicking processes, and systems perspectives. Topics covered include:
-
Engineering of genetic systems for bottom-up SynBio
-
Design and implementation of microfluidic tools for bottom-up SynBio
-
Dynamic protocellular systems
-
Recombinant protein production via cell free expression
Asian players
China: Shenzhen Institute of Synthetic Biology
Established in 2019 as the first institute of SynBio in China, the institute claims to have the world’s largest multidisciplinary team specialized in SynBio.
Singapore: Synthetic Biology for Clinical and Technological Innovation (SynCTI)
Established in 2014 as the focal research program for SynBio at the National University of Singapore (NUS), SynCTI operates as an interdisciplinary research core, interacting with other research programs within NUS and leveraging existing research capabilities in SynBio that have been developed through funding from local and international organizations.
Korea: Korea Advanced Institute of Science and Technology Department of Biological Sciences
The department declares a focus on genomics and nanotechnology, with an emphasis on research on human diseases and their molecular mechanisms, functional genomics and its application, and nano-biotechnologies and their application.
Some key research themes
Looking across the overall scientific landscape, several current SynBio research themes are expected to mature within five to 20 years with the potential for major impact to business and society. Examples include:
-
Wide-scale adoption of cell-free biomanufacturing (high impact, low uncertainty, mature within 5-10 years). The use of cell-free systems for biomanufacturing has the potential to become the new standard in SynBio. Further evolution requires overcoming the challenges of product creation beyond lab scale. Possible applications include the industrial production of green chemicals and novel products across biofuels, cosmetics, and pharmaceuticals.
-
Convergence of AI, SynBio, and automation (high impact, low uncertainty, mature within 5-10 years). Within SynBio, AI offers potential in data mining, bioinformatics, and computational biology, such as for pattern and structure recognition in gene expression data, engineering of biological systems, metabolic engineering, experiment automation, self-driving labs, outcome prediction, and reverse engineering. It offers the potential for step changes in workflow speed, accuracy, and effectiveness.
-
DNA synthesis at genome scale for eukaryotic organisms (high impact, low uncertainty, mature within 5-10 years). New technologies with the ability to synthesize and produce large-scale, complex DNA sequences are under development, including molecular assembly and cloning methods, template-independent enzymatic synthesis, microarray, and rolling circle amplification techniques. Being able to create large DNA sequences at scale and low cost would have major impact on fields as varied as sustainable materials, data storage, drug delivery, and genome engineering to generate organisms with useful properties, such as heat-resistant plants.
-
Fungal SynBio (high impact, medium uncertainty, mature within 5-20 years). Advances in applying biotechnology to mycology and fungal genetics, with advances in long read sequencing, makes it possible to generate high-quality fungal genome assemblies. Fungi have potential in a wide range of applications, such as within environmental remediation, fungal-derived food products, enzyme and other chemical production, sustainable biomaterials, fungal batteries, and many more.
-
3D bioprinting of complex animal and human tissue and organs (high impact, low uncertainty, mature within 10-20 years). 3D-printing technologies can be deployed to assemble multiple cell types, growth factors, and biomaterials. While tissue reconstruction is already feasible, reconstruction of complex organs still has many challenges. For example, heart functionalities like vascularization, mechanical load, and electrical signal propagation must be replicated. If successful, the technology offers the potential for bioartificial organ transplants as well as other medical interventions and treatments.
-
Predictive genetic engineering for multicellular organisms (high impact, high uncertainty, mature within 10-20 years). Despite progress at bacterial and mammalian cell levels, programming of higher organisms is highly complex, requiring, for example, the delivery of gene editing cascades or the engineering of whole embryos. The ability to change the physiology of animals such as worms would open possibilities and applications across agriculture, biomedical, and the environmental domain, notwithstanding potential ethical issues.
-
DNA-based data storage (high impact, medium uncertainty, mature within 10-20 years). Low writing/reading speeds and high costs per byte stored are key challenges for DNA-based data storage technologies. Current research includes using DNA nanostructures (as opposed to DNA sequences) and combinations of nanomaterials and biomolecules. Research has the potential to provide high capacity, long lifespan, and lower energy storage to meet rapidly growing data storage needs.
Tool & technology developers
Tool and technology development can be categorized usefully in terms of the design-build-analyze-enable workflow, essentially a common development engine that is fundamental to all SynBio implementation. The landscape includes large, established players; start-ups; and academia. Figure 12 provides a summary of the main tools and technologies currently in use or under development split across four categories:
-
Design — help model and simulate biological systems
-
Build — assist in construct biological systems
-
Analyze — help test and evaluate biological systems
-
Enable — accelerate the engineering process and, importantly, enable scale-up from lab to industrial production levels
The workflow is similar to that used in conventional non-bio technology development (design, build, test, manufacture), although there are differences. For example, the relative unpredictability of some SynBio processes, and the challenges of scaling up, mean that tools used to analyze and enable are perhaps even more critical.
Tool & technology maturity
Tools have varying maturity levels:
-
Design — generally the least mature (see Figure 13). While core gene and protein design and simulation tools have reached high maturity with well-established key players, the full power of AI and digital technologies remains untapped and will struggle to match demand for mature solutions.
-
Build — generally more mature (see Figure 14). Core technologies such as de novo DNA synthesis and DNA/gene delivery systems are already largely commercialized, while genome-engineering tools (e.g., CRISPR) are still maturing but have high disruptive potential and are on the cusp of wider adoption.
-
Analyze — generally the most mature (see Figure 15). Dominated by a few large players (e.g., Illumina and Thermo Fisher), rising demand for next-generation sequencing (NGS) has helped drive adoption, with the market continuing to grow rapidly.
-
Enable — varying maturity (see Figure 16). Automation and fermentation technologies are fairly mature and established, while cloud labs and microfluidics are low in maturity, with most players being start-ups. However, cloud lab companies are creating strong partnerships with large industry players, indicating the possibility for widespread adoption and growth in the coming years.
Figure 17 shows the relative maturity of selected tools within each of the four SynBio workflow stages, based on ranking by our expert survey panel. Technology readiness levels (TRLs) indicate how close a tool is to proven, commercial adoption.
Market landscape
The majority of high-profile tool and technology players in SynBio are based in America (around half on a per-company basis). The remainder are divided approximately equally between Europe and Asia, with the rest of world currently having few market entrants. The market is split between well over 500 SynBio start-ups and a much smaller number of more established players, with SynBio tool and technology players raising $10.3 billion in 2022. While most top-funded companies are currently headquartered in the US, the most dramatic growth is expected in Asia, led by China.
Many governments have targeted investments and support for SynBio. For example, the UK launched a SynBio program more than a decade ago, while the US, China, and Australia have created their own efforts more recently.
Appendix 1 summarizes selected key players across the four tool and technology development categories.
INTERLUDE #1
Evolving aesthetics — Intersecting art & science in the language of cells
Our explorations in SynBio seek to reveal hidden processes in living matter through the manipulation of genes. We aim to transform these expressions in ways they can be experienced on a human scale.
Consider an E. coli colony growing on a plate. Gradually, it spreads and — as conditions become more demanding — it creates branches and intricate patterns. This phenomenon became the inspiration for our work Stress-o-stat (see first image in this section), which explored how genetic machinery responds to cellular stress. During dire conditions, cells produce the enzyme catalase to neutralize damaging oxygen radicals. Analyzing the genetic mechanisms behind this response made it possible for us to replace the catalase gene with one producing a fluorescent protein. Our new genetic component, embedded in a genetic circuit and inserted into bacteria, caused the emission of an iridescent light during starvation. Using a drip system to regulate food release and a spiral condenser to visualize light changes, we observed the spiral light up during dire conditions and with changing nutrients we saw a slow oscillating light. Stress-o-stat aims to capture the poetic language of “stress” in bacteria, with the luminescence telling a story of resilience and resourcefulness.
To further expand on both method and metaphor, we explored how this could be done using solid media and time-lapse photography. As an additional play on the language of stress and signaling, KatEred (see second image) employed gene-producing, glowing-red fluorescent proteins. Observing growth patterns over time, a blushing red edge glowed as the colony spread across the plate, somewhat akin to tree rings telling the story of time and change. In our micro-environment, the colony creates a living visual story of struggles.
SynBio can be a portal to see processes inside cells, but we can expand on this notation by tapping into other senses like smell. Banana Bacteria (see third image) adds an extra twist by subverting our expectations. It involved the use of a genetic program that could convert an alcohol into banana oil, changing the typical off-putting smell of E.coli into a sweet-scented wonder reminiscent of bananas. It acts as a playful obfuscation that prompts us to introspect our preconceptions.
However, genetically modified organisms are restricted typically to labs; exhibiting outside these settings involves lengthy navigation of health and safety regulations as well as ethics review. Through our work, we have paved the way for the first legal exhibition in showing genetically modified organisms in the UK and beyond its borders.
These works make invisible processes perceptible to humans. Yet, the absence of real-time interaction creates a barrier in how we experience these organisms and processes, often requiring us to add an interface like time-lapse to bridge the experience. Our initial real-time experiments used nanomagnetic particles, which allowed participants to control individual cells using an external magnet.
Surprisingly, we found that magnetic bacteria occur naturally. These organisms swim along the Earth’s magnetic field to optimize their position in a microaerophilic gradient. Exposing these bacteria to a changing magnetic field causes their bodies to reorient and, in doing so, scatter light. In liquid culture, the scattering can be observed as a visible shimmer resulting from blocking or passing light. This extraordinary phenomenon inspired our work Living Mirror (see fourth image). As a mirror, it draws on the idea of water as our original interface predating today’s screen-based technologies and references the myth of Narcissus, who tragically drowned while captivated by his reflection — a reminder of how we continue to immerse ourselves in similar mirrors as we extend our identity into the virtual. To create the mirror, we used a camera to capture a person’s image converting pixels into numerical values. This data activated individual magnetic coils, orchestrating bacteria to produce hundreds of light pulses that formed an image in real time. The work delves into the concept of “self” and how we are predominantly composed of nonhuman bacterial cells. It aims to capture a duality, both technically and conceptual, of how we navigate the intersection of digital and biological perceptions of self.
— C-LAB, an art-science collective founded by Howard Boland and Laura Cinti that combines scientific advancements in the biosciences, critical thinking, and future possibilities through hands-on science
Stress-o-stat
Stress-o-stat is a living artwork that visually captures stress in bacteria as light. The installation combines scientific instruments, methods, and aesthetics to produce a real and experimental device. SynBio tends to postulate a machine-like language onto the living, as something programmable. Paradoxically, it is the opposite of the digital; instead of the machines becoming life-like, it suggests life becoming machine-like.
KatEred
KatEred explores a living visualization of oxidative stress using a glowing-red fluorescent protein and through the use of time-lapse. The colony creates a blushing glow around its edges and creates an imprint of cellular stress over time. Red tends to signify an alert and has been used as “official” semantics in many apparatuses such as warning lights. While these factors play little in a biochemical role, they play with our perception as visual readers.
Banana Bacteria
Banana Bacteria is an olfactory work that explores the paradox of having the foul smell of bacteria exchanged with the sweet smell of banana. These bacteria have been genetically altered by removing a gene responsible for the foul smell commonly found in bacteria and adding a genetic design that enables bacteria to synthetically produce banana oil.
Living Mirror
Living Mirror is a bio-computational imaging system where magnetic bacteria scatter light under a changing magnetic field. This phenomenon was used to create a bio-computational system containing bacteria and individually controlled magnetic coils to recreate patterns and potentially real-time images of people.
3
The applications & industry landscape
SynBio underpins a diverse range of applications across many industries, led by healthcare and life sciences and food and agriculture. The application landscape demonstrates the enormous scope of SynBio. For example, it can be used to create vaccines, improve crops, generate biofuels, and enable DNA-based data storage. Consequently, there are widely varying levels of maturity among applications, ranging from early stage through to full commercialization.
Identifying SynBio opportunities
Our 2023 global expert survey explored industries that would be most impacted by SynBio in the next five to 10 years (see Figure 18). About a quarter of respondents identified healthcare and life sciences as the sector that will see the greatest applicability of SynBio, followed by food and agriculture (21%), and then industrial, manufacturing, chemicals, and materials (18%).
Application maturity
Mapping selected SynBio applications against the Gartner hype cycle further demonstrates both their range and the diversity of their maturity levels (see Figure 19):
-
Healthcare and life sciences. Various subsectors in healthcare are at differing points on the curve. For example, widely used monoclonal antibodies as a treatment option are at the peak of productivity, whereas newer therapeutic modalities like gene therapies are considered to be at the peak of inflated expectations.
-
Food and agriculture. This sector is approaching the peak of inflated expectations, as it is maturing with products that are commercially available, yet market uptake is still relatively limited. Newer technologies that use SynBio techniques are on the brink of commercialization and those that address current sustainability challenges around food production (e.g., cell agriculture for cultured meat) are at the technology trigger stage.
-
Industrial, manufacturing, chemicals, and materials. This segment is approaching the peak of inflated expectations, as demand for novel enzymes for many end-use industries continues to grow. Commodity chemicals are also at the peak of an inflated expectations phase, while partnerships between industry players (e.g., Unilever) and SynBio companies continue to form.
-
Consumer products. Approaching the slope of enlightenment, this segment is maturing with some commercially available products (e.g., fragrances, plant-based leather alternatives). With sustainability at the forefront of consumers’ minds, the use of SynBio to generate consumer products will continue to grow.
-
Energy. This sector is at the trough of disillusionment, as biofuels and bio batteries continue to be slow to overcome economic and technical barriers. However, next-generation biofuels produced from waste materials and other co-products will still have applications in, for example, heavy-duty transport, such as aviation and shipping, that are difficult or impossible to electrify.
-
IT and technology. Just beyond the technology trigger phase, major players are starting to investigate the adoption of SynBio tools and techniques like DNA data storage. This is evident through the growing number of partnerships between SynBio companies and tech players (e.g., Twist and Microsoft; Illumina and Dell).
Key areas for growth
Comparing growth and maturity provides an indication of the significance of each of the key application sectors today (see Figure 20).
As Figure 20 illustrates, healthcare and life sciences is the most mature and largest market, with one of the highest growth rates. Food and agriculture is next, although there is still extensive tech development in this segment at low TRLs. Industrial/manufacturing/chemical/materials, consumer goods, and energy are also growing, although barriers toward commercialization are higher, with IT and tech being the most embryonic. However, if DNA-based storage could be commercialized, this could become a huge market.
The remainder of this chapter takes an in-depth look at each of these markets, including potential applications, opportunities, and challenges.
Healthcare & life sciences
Motivations for SynBio applications in the healthcare and life sciences sector are strong. Key catalysts include:
-
Rising incidence of chronic and debilitating disorders (e.g., cancer). The aging population and resulting rise in chronic diseases increases the need for more specialized treatments.
-
Shift toward personalized/precision medicine. The movement toward more targeted, individualized treatments/therapeutics will increase SynBio use, as it enables fine-tuning of organisms to perform specific/dynamic functions.
-
Limitations of current treatments. SynBio can improve both efficacy and safety of current treatments, as well as satisfy the need for a new treatment method and mechanism.
However, success will require the sector to overcome existing challenges in four key areas:
-
Regulations. Strict regulations regarding drug development and a relatively untested regulatory environment make the process lengthy and costly.
-
Ethical limitations. Public hesitancy and debates regarding ethics of modifying the genome hinder SynBio expansion.
-
Limited technological capacity (e.g., data streams and computer capacity). Technological capacity limitations hamper the ability to actualize all possible SynBio developments.
-
Complexity of large-scale production and manufacturing of cell therapies. The complexity around manufacturing cell therapies and the limitations of large-scale production complicate the process of taking a SynBio concept from lab to commercial scale.
Application areas (non-exhaustive)
Tissue engineering
SynBio enables the creation of multicellular structures for regenerative medicine, drug testing, and beyond, including tissues and organs using cells and structural components to mimic the morphology and function of native tissues.
For example, gene therapy, advanced biologics, and small molecules are being studied to stimulate the regeneration of damaged heart cells. SynBio can be used to generate tissues that can then be transplanted. As an example, cultured epidermal autografts are being used as a permanent skin replacement for the treatment of patients with serous burn injuries.
The tissue engineering market is already large and well established at around $15 billion globally in 2022, with growth of more than 15% CAGR to 2030. Most SynBio applications are still at foundational stages, with key players like Integra LifeSciences and C.R. Bard (acquired by BD in 2017) still focusing mainly on conventional technologies. Start-ups such as Organovo focus on new 3D biomaterial printing.
Medical devices & implants
Integrating SynBio into wearables could expand opportunities for noninvasive monitoring of physiological status, disease states, and exposure to pathogens or toxins to practically enable biosensors. However, sustaining living organisms in medical devices for extended periods remains a challenge.
Potential application examples include new wearable or implanted medical devices for health monitoring and control. Demonstrating this trend, a prototype face mask with a lyophilized (i.e., freeze-dried) CRISPR sensor has been developed for wearable noninvasive detection of SARS-CoV-2 at room temperature.
Vaccines
Vaccines are commonly prepared from an inactivated or weakened form of the causative agent or from its constituents. SynBio facilitates shorter development cycles through alternative technologies, such as nucleic acid vaccines (e.g., mRNA), viral vector vaccines, or recombinant vaccines (e.g., SHINGRIX).
For example, synthetic RNA platforms allow for rapid, scalable, and cell-free manufacturing of prophylactic and therapeutic vaccines. The Pfizer-BioNTech COVID-19 RNA vaccine was the first to be approved for humans. Moderna and BioNTech are examples of key players that used synthetic messenger RNA to protect against COVID-19.
Stem cell therapies
Stem cells serve as the raw material from which all specialized cells are derived, capable of generating healthy replacements for cells impacted by disease (regenerative medicine). Achieving this transformative process is made possible through genetic reprogramming techniques such as CRISPR. For example, stem cells can be manipulated into heart muscle cells, which can then be injected into the heart muscle of a patient with heart disease. The transplanted cells would then aid in repairing the injured muscle.
The global stem cell therapy market was valued at ~$10 billion to $12 billion in 2022 and is predicted to grow at a 10%-15% CAGR for the next 10 years. An influx of interest and funding has resulted in both emerging players, like bluebird bio and BlueRock Therapeutics, and more established players, like Mesoblast and Osiris Therapeutics, coexisting in the expanding market.
Cell therapies
SynBio enables the development of therapies based on bioengineered cells. In these currently highly personalized treatments, cells are becoming the treatment modality. Among the first treatments in this space was Kite Pharma’s CAR T-cell therapy, but there are many more in development to treat a variety of disorders and diseases. For example, CAR T-cells have successfully treated blood tumors such as large B-cell lymphoma and also promise a new scenario of therapeutic interventions for solid tumors.
The genetically modified cell therapy market is projected to grow rapidly from $2.7 billion in 2022 to $32.3 billion in 2029 at a CAGR of 42%. Main players in the space include large pharma players such as Bristol Myers Squibb and Gilead, which have expanded their cell therapy capabilities through acquisitions of Juno/Celgene and Kite Pharma, respectively. However, there are several smaller biotech players harnessing this technology with assets in the early development phases, including Allogene and Ixaka (formerly Rexgenero).
Gene therapies
SynBio has enabled scientists to cure diseases by correcting the underlying genetic defects through genomic editing (e.g., CRISPR) or introduction through a vector (usually a viral vector). Alternatively, synthetic oligonucleotides can be used to modify the genetic response, with RNAi currently being the most prominent technology. For example, vector-based gene therapies have been approved for both hemophilia A and B. In both cases, viral vectors are used to deliver the correct gene and potentially cure the patients through a single treatment, although long-term data is not yet available.
The gene therapy market is projected to grow rapidly at a CAGR of ~20% from $7.5 billion in 2022 to ~$30 billion in 2030. Examples of key established players are large pharma players such as Novartis as well as more niche players like Vertex. The approved gene therapies are currently centered on hematological genetic diseases, such as hemophilia and severe sickle cell anemia, but also include degenerative inherited diseases. Gene therapies for some larger indication areas, such as type 1 diabetes, are also in development.
Bio-machine interfaces
SynBio technology could provide solutions for developing effective bio-human-machine and neuro-machine interfaces. For example, it has been demonstrated that closed-loop brain-machine interface (BMI) technology can modulate sensory-affective experiences in rats, illustrating how a BMI approach could be used for pain control as an alternative to drugs. Numerous healthcare applications exist, such as restoring physical functions and controlling symptoms. There are also applications in education and entertainment, where BMI technology could be used, for example, to control avatars and objects, contributing to a more immersive gaming experience.
Maturity timeline
In the future, SynBio is expected to lead to more sophisticated treatments, greater safety from more diseases, and new applications for existing conditions, such as neurological and genetic ailments. Figure 21 outlines the maturity timeline across the healthcare and life sciences sector.
Food & agriculture
Feeding a growing global population while coping with the immediate impacts of climate change is a critical challenge for the world for several reasons:
-
Sustainability and accompanying policy and regulatory changes. Demand is growing for SynBio solutions due to their ability to provide results while protecting the environment and maintaining or improving soil fertility.
-
Population growth. Population growth has been projected to require improvements in productivity/yield by 60%-80% up to 2050, increasing demand for SynBio improvements. Initiatives are increasingly being driven by governments (e.g., Singapore’s 30 by 30 food security and sustainability plan).
-
Plateauing yields with current improvements. Limitations in current farming improvements, such as fertilizers and greenhouses, are making the industry look to other more effective options for higher yields.
Successful SynBio adoption will require the sector to overcome existing challenges in three key areas:
-
Regulation. Elements of the emerging regulatory environment remain untested, creating compliance challenges.
-
Public perception. Consumers, especially in Europe, have negative views of existing genetically modified food and technologies (e.g., gene/genome editing). This negatively impacts demand for SynBio-derived products and potential market growth.
-
Risk aversion. SynBio adoption involves disruptive change within the market. Larger companies with established systems, in particular, are reluctant to change how they operate, holding back adoption.
Application areas (non-exhaustive)
Crop domestication & breeding
SynBio enables metabolic pathways, genetic circuits, and plant architectures to be engineered for agricultural plants, enhancing their suitability to human needs, such as around taste/flavor, color, yield, storage, productivity, amino acid production, ability to fix nitrogen, and disease resistance. SynBio offers a major enabler to reduce the time required for crop breeding cycles by making use of existing genomic data sets, as well as deploying other toolsets to expedite the breeding cycle which, for some crop varieties, may take five to seven years. For example, Pivot Bio used an engineered bacterium to create a biological nitrogen fertilizer for corn, reducing the need for chemical fertilizer while increasing yield.
The crop-breeding market is expected to reach over $30 billion by 2029. Major players, such as Benson Hill and Apeel, are being joined by smaller companies like Tropic Biosciences.
Precision agriculture
SynBio can improve the physical properties of soil in a variety of ways; for example, through improving soil microbiomes via microbial fertilizers, enabling soil remediation, and developing natural pesticides, as well as helping to diagnose potential pests and diseases in soil and water. This type of improvement includes the use of novel bio-fungicidal seed treatments to control soil-borne fungal pathogens that cause seed rot, damping-off, root rot, and seedling blights while minimizing environmental impact.
The global precision agriculture market reached a value of around $6.7 billion in 2021. Governments across the globe have undertaken several initiatives to encourage the uptake of modern agricultural techniques to enhance crop yield and productivity. Syngenta Group and Bayer Crop Science are leaders in precision agriculture. However, as the need for precision agriculture continues to grow, there are several start-ups and smaller players entering this space, such as Pivot Bio, Concentric, and Robigo.
Designer synthetic & bio-based food
SynBio can be applied to engineer food not produced in natural soil or obtained from animals (e.g., microbial nutritional protein). Such food is produced artificially in both laboratory and industrial conditions, using a culture or growth medium. For example, in 2016, Impossible Foods launched the Impossible Burger, a vegan alternative to a beef hamburger. The process uses genetically engineered yeast to produce the heme protein needed to make the food look and taste more like meat.
The global synthetic food and ingredients market is estimated at around $12 billion to $13 billion in 2022. Impossible Foods and Beyond Meat currently dominate the plant-based meat market. The increasing number of new plant-based food start-ups and the expansion of the food service industry will further drive growth. Start-ups providing animal-free dairy proteins, including ImaginDairy and Perfect Day, are also rising in popularity.
Bioremediation, biosequestration, biosensors
SynBio can be used to create genetic-engineered microorganisms and microbial consortia to improve the efficiency of bioremediation, especially in heavily contaminated or difficult-to-access environments. An example of this is the work of UK-based CustoMem, which uses SynBio to create a granular material that attracts and sticks to micropollutants, such as pesticides, pharmaceuticals, and certain chemicals in wastewater.
The global bioremediation market is estimated at around $13 billion to $14 billion in 2022. Growth will be driven by increasing environmental awareness as well as regulatory demands, with bio alternatives perceived as more natural, as well as being cost-effective in many cases. The market is fairly fragmented, but major players include Xylem, Drylet, Aquatech, and others.
Maturity timeline
Moving forward, we expect SynBio adoption to lead to further developments in crop technologies, food engineering, and environmental remediation approaches across the food and agriculture sector (see Figure 22).
Industrial, manufacturing, chemicals & materials
The need to decarbonize, reduce waste, and become more sustainable is increasingly impacting industrial companies and their operations. Key drivers include:
-
Sustainability needs and accompanying policy changes. Increased global awareness of environmental issues will likely result in further regulations restricting fossil fuel manufacture.
-
Technological advances enabling new innovations. New and evolving technology will reduce the cost and complexity of applying SynBio developments.
-
Waste reduction from large-scale production. SynBio technologies can both improve and replace current manufacturing processes that produce large amounts of waste.
-
Rare earth and mined materials supply constraints and security. The transition to net zero will require huge increases in mined raw materials, especially metals. This will increase further the need for more sustainable production processes.
However, major challenges to SynBio adoption remain in three key areas:
-
Cost. Though more sustainable, the high cost and greater energy consumption of SynBio processes at scale are major barriers to adoption.
-
Risk aversion. Players with large-scale, established infrastructures (e.g., chemicals industry) and profitable systems are hesitant to adopt SynBio alternatives, due to the high costs and risks inherent in such a major transformation.
-
Collaboration. Many SynBio solutions require the formation of new ecosystems, creating nontraditional partnerships; for example, between materials companies, technology providers, manufacturers, consumers, and environmental companies.
Application areas (non-exhaustive)
Bio-based specialty products
Bio-based specialty products can be created via new production pathways where microorganisms generate a wide variety of chemicals. While many processes are already established, SynBio offers the potential to improve them. For example, the hyaline family of clear, flexible films are used for flexible electronics (e.g., foldable smartphones and wearable electronics).
The bio-based specialty products sector is primarily dominated by larger players, including BASF, Toray Industries, and Novamont. These companies have significant financial backing and partnerships already in place. Companies like Toray have developed plant-based synthetic fibers, while Novamont has focused on the development of biodegradable bioplastics by applying SynBio techniques.
Sustainable production/manufacturing
Using microorganism strains engineered to produce defined outputs (e.g., production of small molecules of high value) helps reduce reliance on petrol-based and even plant-based chemicals and processes. For example, engineered microbes and biotechnological copper-recovery applications are already being applied in copper mining, including for bioleaching, accumulation, and recycling.
The market for sustainable production/manufacturing is extremely broad and diverse, including, for example, pharmaceuticals, commodity chemicals and materials, consumer goods, and bioremediation, all of which are covered elsewhere in this Report.
Commodity chemicals & materials
Commodity chemicals can now be engineered and produced from microbes and more renewable materials (e.g., value-added chemicals can be synthesized from flue off-gases). As one example, Biomason uses bioengineered bacteria to “grow” bricks. The company claims these “Biocement” bricks are as strong as traditional cement and are made on-site using local materials.
Biotech companies such as Ginkgo Bioworks and Genomatica have focused on the commodity chemicals and materials sector, with Gingko valued at $15 billion in 2021. Genomatica has developed partnerships with Unilever to develop alternatives to palm oil and fossil fuels. The company has also partnered with global chemical company Asahi Kasei to commercialize renewably sourced nylon.
Enzyme production
SynBio techniques such as DNA synthesis and heterologous expression can be used to produce novel enzymes with improved properties. For example, Arzeda has developed Intelligent Protein Design Technology, which combines physics-based protein design and AI to create new designer proteins and enzymes, which it claims to be more efficient and less risky than conventional approaches.
The rising demand for novel enzymes is attributed to growing product demand in various end-use industries (e.g., detergents for industrial applications). Established players like Novozymes and BASF have several partnerships in place with companies across consumer care and biopharmaceuticals. Additionally, there are many less mature players, including Arzeda, that have received limited funding but have developed significant partnerships with companies, including Unilever, Amyris, and BP.
Maturity timeline
In the future, we expect increased penetration of SynBio technologies into industry and manufacturing, provided that the economic challenges can be solved (see Figure 23).
Consumer goods
Sustainability and personalization, particularly in the luxury goods and fashion sectors, are strong drivers, in addition to the following:
-
Sustainability and accompanying policy changes. SynBio solutions typically offer sustainability benefits for governments looking to meet sustainability goals.
-
Consumer awareness. There is increasing consumer awareness and demand for more sustainable production and products.
-
Personalization. There is a growing market for designer, personalized, and high-end consumer products. Personalization is a major trend in luxury consumer goods.
-
Rising energy prices. High energy prices are driving manufacturers to seek alternative processes and less energy-intensive production processes, perhaps even at the expense of time-to-product.
Successful SynBio adoption will require the sector to overcome existing challenges in three key areas:
-
Scale. Many consumer products using SynBio can only be produced on a small scale. The cost and time-to-market for these products may therefore be very high, especially given that some involve relatively novel technologies.
-
Cost. The cost-effectiveness and established infrastructure for fossil fuels in commercial production can make changing to SynBio economically unattractive, particularly as it is a less mature technology.
-
Public perception. Consumers may be wary of SynBio products, particularly in areas where “natural” products are seen as premium, or where humans are in close physical contact with the product (e.g., cosmetics).
Application areas (non-exhaustive)
Flavors & fragrances
By applying biosynthesis and precision fermentation, SynBio creates alternative pathways for molecules traditionally sourced from plant or animal materials. For example, Evolva applies precision fermentation to develop flavors and fragrances, such as nootkatone, valencene, vanillin, and L-arabinose.
The global fragrances market has been valued at $59 billion in 2023. Both Amyris and Conagen are mature players in the SynBio-based fragrances space. Amyris has had several partnerships to develop bio-based fragrances, including with Maison Francis Kurkdjian. Conagen is a global leader in developing natural fragrances and its vertical integration has enabled it to rapidly commercialize fragrances.
Consumer materials, textiles, fabrics
SynBio provides the ability to create more sustainable (carbon-negative) textile materials, both improving existing textiles and creating novel, animal-free materials. For example, Hide Biotech produces a leather-like material by transforming food industry waste into a customizable collagen-based leather substitute.
The global vegan fashion market, which includes any apparel not using animal products including non-synthetic materials, was valued at around $450 billion in 2021 and is expected to grow at a CAGR of 7.1%. While there are several small players entering this space, more established players like Bolt Threads have established strong partnerships with the likes of Adidas and Stella McCartney. Genomatica has developed a novel plant-based nylon and has recently partnered with lululemon to use its sustainable fabric. Most players in this space are focused on developing alternative materials to offer consumers more sustainable choices.
Consumer care products
SynBio can be applied to develop ingredients and compounds for skincare and beauty products, replacing less sustainable production processes and moving away from animal-derived products. As an example, Biossance produces skincare products based on a skin-identical hydrating molecule, squalene, made from sustainable fermented sugarcane.
There are a several SynBio companies with commercialized products in the consumer care space. Companies like Amyris have successfully developed trans-β-Farnesene-derived squalene, which is used across several personal care products today. As another example, haircare company K18 has patented K18PEPTIDE, a high-performance ingredient that reverses hair damage at the molecular level. Since its launch, the product has seen significant consumer adoption.
Consumer healthcare & wellness
Ingredients for health and nutritional products can be made from more renewable alternatives through SynBio. For example, Ayana Bio has launched lemon balm and echinacea products made via plant cell culture, which it claims can provide a consistent supply of botanicals without the complexities of existing supply chains.
The largest player in the consumer healthcare and wellness space that leverages SynBio is Conagen, which has recently developed resveratrol made by clean bioconversion. Other players with commercialized products include ZBiotics, which offers a product that helps the gut break down acetaldehyde, and Sun Genomics, which produces custom probiotics.
Maturity timeline
Moving forward, we expect wider ranges of SynBio-produced consumer goods that offer greater personalization and tailoring (see Figure 24).
Energy
Energy players have to decarbonize to meet increasing regulations and net zero targets while ensuring energy security and coping with the impacts of geopolitical uncertainty. As such, the relevant drivers for SynBio solutions include:
-
Sustainability and accompanying policy changes. Fluctuations and potential increases in fuel prices driven by introduction of carbon taxes, scaling back of oil production, and geopolitical uncertainty could make bio-based routes more attractive.
-
Energy insecurity. This is driving a significant push to move away from depleting fossil fuels, resulting in increasing demand and willingness to pay for SynBio fuels.
-
Incremental biofuel uses. Bio-based fuels may add incremental value in extreme weather conditions or specific applications, such as aviation and marine fuels, where electrification is not a viable option.
Successful SynBio adoption will require the sector to overcome existing challenges in three key areas:
-
Cost. Biofuels are generally much more costly than fossil fuels, and there are often major raw material logistical challenges. The established systems and infrastructure of fossil fuel use, and huge upfront investments required for conversion, hinders the establishment of biofuels as cost-effective options on the market.
-
Resources. Despite innovations to date, current biofuel production technologies do not consistently deliver the volumes required for commercial production, resulting in inefficiencies in scale-up.
-
Electrification. A shift toward electricity for mobility implies an overall decline in oil use, even including bio-based alternatives. This uncertainty further hinders investment decisions.
Application areas (non-exhaustive)
Biofuels
Organisms can be engineered using SynBio to produce biofuels from waste materials or biomass feedstocks rather than food crops, as well as to produce novel fuel molecules with better performance/higher energy content than current fossil fuel alternatives. For example, the metabolic engineering of yeasts is enabling microbial cell factories for biofuel production.
The second-generation biofuels market — comprising fuels produced from waste materials rather than food crops — was valued at $6 billion in 2020 and is projected to reach $59.4 billion by 2030. Today, this comprises mostly hydrogenated waste vegetable oil produced by Neste and some long-established smaller businesses seeking to use genetically engineered microorganisms for advanced fermentation. Companies such as Poet have tried to make this technology work at scale for many years but have faced significant headwinds, mainly due to feedstock availability and pre-treatment issues.
Bioelectricity & bio-batteries
SynBio techniques can be used to create engineered organisms that produce “cell factories” capable of generating energy from organic materials. These include organisms capable of producing bioelectricity and photosynthetic biomaterials for solar panels, for example. Bio-batteries are distinct from microbial fuel cells, which produce electricity from the microbial oxidation of reduced compounds. As an example in this area, scientists at the UK’s Defence Science and Technology Laboratory are researching a bio-battery based on DNA technology that will be much safer in the case of destruction during conflict.
Microbial fuels cells have been under development for many decades, but their application has been limited due to high electrode costs and low power. Bio-batteries, which are based on the same principles as human/animal digestion, are still in their infancy. Recent examples involve battery concepts based on lactates with enzymes bound to a structural scaffold.
Bioremediation & biosequestration for energy
SynBio can be used to improve processes for waste treatment, such as by speeding up or enabling natural decomposition. These methods can be used to treat waste in situ, such as following an oil spill (especially on land), while also having the theoretical potential to convert waste to energy, though processes are extremely slow and inefficient today. For example, LanzaTech’s carbon recycling uses engineered bacteria to convert pollution to fuels and chemicals.
The global bioremediation market in 2022 is thought to be worth around $13 billion, with most forecasts suggesting growth of 9% CAGR to around $30 billion in 2030. Metabolic engineering, enzyme modification, and other SynBio technologies are key enablers. Bio-based routes to carbon sequestration through the capture of flue gases continue to be largely economically unviable, although players are making progress in this area, with LanzaTech currently leading the field.
SynBio for hydrocarbon extraction
SynBio techniques can improve the extraction of traditional hydrocarbon or fossil fuels, such as through enhanced oil recovery techniques (EOR) to help process more difficult-to-extract reserves from depleted oil wells. Today, the use of SynBio in EOR is still an emergent technology that is relatively slow and inefficient and has not yet been proven at scale. However, recent lab-scale research is showing progress. For example, a recent study published by the American Chemical Society has shown that biosynthesized nanoparticles can be used to increase oil recovery rates by several times.[4]
Maturity timeline
Moving forward, we expect to see further improvements in biofuel viability as well as other solutions, including electricity and the circular economy (see Figure 25).
IT & technology
In an increasingly digital world, mankind is producing, storing, accessing, and analyzing progressively larger amounts of data. Volumes are growing exponentially, pointing to future needs for new forms of data storage, cybersecurity, and novel computing paradigms that can solve currently intractable problems.
While still in its infancy, DNA data storage could ultimately provide much higher storage density and ease of synthesis, while biocomputation and bio-inspired cryptography offer future advantages over current methods.
In IT, SynBio applications are still at the PoC stage and must overcome at least five key challenges:
-
Immaturity. Error rates in DNA computing need to improve to allow biocomputing and cryptography.
-
Technical limitations. The capabilities within encoding, writing, storage, reading, decoding, and so on, render DNA storage currently uncompetitive with magnetic and solid-state storage devices.
-
Cost and throughput barriers. Despite theoretical advances in lower-cost synthesis techniques, commercial synthesis costs have not changed drastically, limiting large applications. Additionally, current DNA sequencing throughput means intended applications are uncompetitive.
-
Slow commercialization. There is a lack of current initiatives to turn lab research into applications accessible by end users, hindering rapid commercial adoption.
-
Competition. Extensive development is taking place using a range of other computational and data management technologies with promising results in some areas.
Application areas (non-exhaustive)
Data storage
By nature, DNA is used to store genetic information. However, it can also be used as a storage replacement for magnetic and optical media. DNA can store data for over 500 years with little to no data loss and is extremely dense, holding up to 1 exabyte per gram. Data can also potentially be stored inside living cells, opening up applications dubbed DNA of Things (DoT). For example, start-up player Catalog claims that its current Shannon prototype computation and storage platform has demonstrated PoC.
As far back as 2015, Microsoft invested in a project with the University of Washington, USA, to develop molecular-level data storage. And in 2020, Illumina, Microsoft, Twist Bioscience, and Western Digital created the DNA Data Storage Alliance to build an interoperable storage ecosystem based on DNA as a data storage medium.
Biocomputation
Biocomputers use biologically derived materials to perform computational functions. Types include biochemical, biomechanical, and bioelectronic computers. In theory, their abilities to self-replicate and self-assemble could lead to low-cost production in the future, while they may ultimately have the potential to deal with “intractable” problems that are hard to solve via conventional computers. Biocomputation is still at an embryonic stage. While examples have been created that can perform simple binary logic calculations, an engineered biocomputer is thought to be at least 20+ years away.
Today, biocomputation is driven mainly by universities, like the Centre for Biotechnology and Plant Genomics at Technical University of Madrid, Spain, and a few start-ups, such as Catalog. Additionally, companies such as Biocomputation Lab offer biocomputation services (e.g., designing biocircuits to perform predefined functions, mathematical modeling, and computational simulations to understand biological systems).
Cryptography
Bio-inspired cryptography is an emerging approach leveraging the high storage information density and parallelism of DNA. While it has been used for encryption and decryption algorithms, it is still at an embryonic stage, with no current examples of its deployment. Research institutes and universities like the University of Potsdam, Germany, are engaged in developing a molecular labeling system with position-oriented DNA encryption. Catalog’s claimed DNA-based computation device should also cover cryptography.
Maturity timeline
Full-scale DNA-based data storage systems and DoT applications are estimated to be at least 10 years away (see Figure 26).
INTERLUDE #2
Philosophical & ethical issues surrounding SynBio
SynBio has piqued the interest of philosophers and ethicists, leading to a cross-fertilization among various subfields of philosophy, including philosophy of science, branches of applied ethics, as well as philosophy of technology and areas of the social sciences that address questions about responsible research and innovation — and the broader relationship between science and society. Researchers in these domains are working to clarify relevant concepts in relation to specific areas of SynBio. Protocell research, for example, has sparked philosophical debates about the explanation and definition of living systems and the concept of emergence. Targeted mutagenesis in plants has stimulated thinking about the definition and ethical relevance of “naturalness”: Is a plant that is a result of targeted mutagenesis still a natural object, is it a technological one, or is it a kind of hybrid? For whom does it matter?
Conceptual clarification like this can help inform normative questions pertaining to the right course of action. For example, to what extent should we be concerned about our abilities to intervene intentionally in the most basic and fundamental of vital processes, often referred to as “playing God”? What is the best way to govern the complex relationship between research, government, and private interests in SynBio? How can we ensure that risks emerging from technologies that can intervene in the basic processes of life to create new functionalities or even new organisms are managed without obstructing their potential to create various forms of value?
These questions are multifaceted. The concern about “playing God,” for instance, takes two main forms: one is primarily ethical and concerns the normative limits of instrumental human control over the natural world; the second relates to whether public concerns about synthetic biologists playing God might impede progress in the field. In the first case, philosophers have pointed out that there is nothing novel about humans instrumentalizing other forms of life and trying to exert control over evolution and development (with agriculture being a primary example).
The second concern, relating to public attitudes toward science and democratic governance of science, was somewhat quashed by public consultations demonstrating that public concerns about SynBio relate more to questions pertaining to the distribution of benefits and risks: who controls these new technologies and to what end? — traditional political questions. These attitudes toward SynBio track earlier reports of public perceptions of similar technologies, such as GMOs in agricultural biotechnology.
From a sociopolitical perspective, SynBio has stimulated discussion about the governance of “technoscience,” forms of scientific activity requiring sophisticated technical infrastructure that necessitate large-scale public or private investment. In this way, SynBio has been an excellent case study for discussion on the role of democratic decision-making mechanisms in science and research governance and the implementation of responsible research and innovation in publicly funded research programs.
Overall, SynBio continues to require ethical and political guidance but also stimulates new pathways of inquiry for classical questions in philosophy, ethics, and politics.
— Darian Meacham, Maastricht University
Darian Meacham is Chair of the Philosophy Department at Maastricht University, the Netherlands. He is also the Principal Investigator for Ethics and Responsible Innovation at the Brightlands Institute for Smart Society (BISS). From 2015–2019, he worked at BrisSynBio, a multidisciplinary research center based at the University of Bristol, which focuses on the biomolecular design and engineering aspects of synthetic biology.
4
How companies should respond
A wide range of companies could see their current or future businesses impacted by SynBio, including:
-
Companies that have relevant capabilities for the current/future SynBio design-build-analyze-enable workflow
-
Companies in application sectors such as healthcare and life sciences, food and agriculture, industrial/manufacturing, consumer goods energy, or IT and technology
-
Investors who may be considering taking a position in respect of actors in the SynBio value chain
These organizations should consider four steps to assess how SynBio could impact their business (see Figure 27).
Identify potential opportunities
Identifying opportunities must be both systematic and creative. A good starting point is to understand the areas of the business that could be disrupted, or where new opportunities could be created, by current or envisaged future SynBio technologies.
For example, the taxonomy outlined earlier in Figure 4 is a guide to finding opportunities where SynBio could enable:
-
Creation of novel products or use of new/different raw materials
-
Enhanced performance of existing products, either directly or through substitution
-
Improved sustainability of existing production processes
-
Greater process efficiency, better precision, or more customization in production and manufacturing
As always, combining a systematic product/process review together with open-ended what-if exercises is likely to produce optimal results.
Assess attractiveness & develop strategy
Even with early-stage applications or those that are not yet mature, it is important to consider the potential business case. This is especially true in SynBio, which often has been characterized by a “solutions looking for a market” philosophy. It is therefore important to take a market-led, business model approach, considering the barriers that need to be overcome to move from viable product to full commercialization (see Chapter 3 for more details on these barriers).
Given the difficulties and challenges of scaling production, it is likely that high value-add-niche SynBio applications will often be more attractive than those where the business model requires high-volume production.
It is valuable for companies to outline a strategy — even if at only a high level. Ideally, the strategy should be scenario-based to reflect critical uncertainties like skills/funding/investment availability, public perception/ethics, and overcoming scale-up challenges. It should include SynBio status, objectives, priorities, programs, and a roadmap to monitor progress.
Depending on the range of opportunities identified, a portfolio approach can be valuable, as it provides a balanced program of projects to counteract the challenges and potential long-term nature before SynBio opportunities deliver results.
Develop capabilities
SynBio is a multidisciplinary field and requires many specialist capabilities. It is extremely unlikely that any one player will have all these capabilities and skills in-house, so understanding the player landscape is essential.
Once a company has selected particular opportunity areas, it should engage with relevant key players in the partner ecosystem. Understand that for many application areas the ecosystem is extensive, including, for example, enabling players as well as toolset and product developers. This calls for thorough research and due diligence.
In conjunction with ecosystem collaboration, players should establish relevant in-house capabilities. What these are and the depth of skills required clearly depend on the size of any investment, ranging from “intelligent buyer” at one end of the scale through to requiring full, in-house expertise at the other.
Build & maintain ecosystem presence
As always with developing technologies, the timing of growth rates and innovation breakthroughs are difficult to predict. Involvement and presence in the ecosystem are therefore key to responding rapidly if and when an application starts to see exponential growth. SynBio has shown recent accelerations that suggest it could very well achieve this exponential growth in the coming years.
Involvement in the ecosystem is also important to engage constructively in discourse around key ethical and regulatory issues.
Generally, application end users will have invaluable expertise and knowledge to offer in respect to markets, customers, and go-to-market strategies. This expertise is especially useful for technology and product developers, many of which will be start-ups and may come from an academic or technical perspective.
Conclusion — Companies need to be ready for growth
Overall, the SynBio market faces significant challenges to success. To better understand these, our 2023 global expert survey ranked known SynBio challenges in terms of their importance/criticality and the level of uncertainty around whether they would be overcome in five to 10 years (see Figure 28).
Survey responses show a large number of challenges that combine high criticality and high uncertainty, including:
-
Overcoming key skills and competency gaps (including genetic-editing capabilities)
-
Achieving standardized toolsets and systematizing the knowledge base
-
Continued availability of funding and investment
-
Negative public perception and overcoming ethical and biosecurity concerns
-
Meeting the challenges of scaling up for industrial-level manufacturing
-
The availability of big (biological) data
- The challenge of better DNA synthesis tools (#7) was also considered critical but was considered more likely to be overcome within the five- to 10-year time frame. Other important challenges with high uncertainty included:
-
IP/technology ownership challenges
-
Overcoming fundamental technology and scientific challenges
-
Regulation and policy barriers
-
Insufficient market demand (due to poor economics)
-
Lack of coordinated programs and open innovation
- Compute power and speed of simulations (#13) is also an important enabler but was judged more likely to be achieved within the time frame.
In conclusion, the challenges to the successful growth of the SynBio market are significant and wide ranging. There are many critical uncertainties and, in many SynBio applications, development has been ongoing for decades, often driven more by technology push than market pull.
However, the potential SynBio offers is equally strong, and companies should understand that:
-
SynBio is an extremely diverse field. SynBio is fairly unique in the breadth of potential applications — from healthcare to food, to manufacturing, to industrial processing, and even to the digital industries. Its technologies range from the embryonic through to the fully commercialized. In considering its prospects, it is therefore important to take a sufficiently granular approach, based on the field.
-
Many SynBio applications are poised for growth. Forecast growth rates for SynBio are >20% CAGR to 2030 overall, especially in healthcare and life sciences and food and agriculture. Drivers for growth are strong, both on the supply side (increasing investment, lower technology costs, growing ecosystems) and the demand side (e.g., sustainability, healthcare needs, manufacturing efficiency).
-
SynBio technologies could have a massive transformational impact in a 20-year time frame. Technologies such as predictive genetic engineering, DNA synthesis for complex organisms, DNA-based storage, and AI-powered applications could transform industries, our ability to engineer living systems, and how we cope with climate change.
All this means that companies should ensure they have a suitable SynBio strategy in place. Given its diversity, companies must consider carefully which specific fields are relevant for their business and conduct realistic market-based assessments. At the same time, due to the strong drivers, accelerating technology developments, and potentially huge impacts, companies should be prepared for exponential growth that could result from breakthroughs as we move toward a SynBio world.
Appendix 1
Example SynBio tool & technology players
Appendix 2
Example SynBio application players
Notes
[1] Gallagher, James. “Scientists Grow Whole Model of Human Embryo, Without Sperm or Egg.” BBC News, 6 September 2023.
[2] Survey comprised responses from 38 academic and industry leaders from around the world.
[3] Hybrid: systems that combine organic and inorganic materials.
[4] Wang, Bo, et al. “Synthesis and Enhanced Oil Recovery Potential of the Bio-Nano-Oil Displacement System.” ACS Omega, Vol. 8, No. 19, May 2023.
DOWNLOAD THE FULL REPORT
57 min read • Healthcare & Life Sciences
The brave new world of synthetic biology
Major impacts, significant challenges
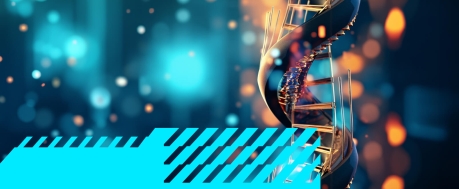
DATE
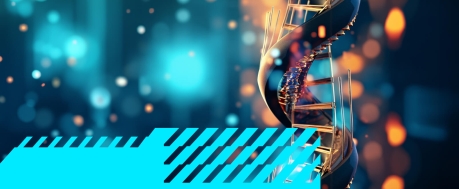
Executive Summary
“What I cannot create, I do not understand.”
— Richard Feynman, theoretical physicist
While the 20th century was in many ways the century of the industrialization of chemistry, the 21st century is promising a similar revolution in biology. The revolution’s foundation began with the discovery of DNA in the 1950s and expanded to core technologies that help to understand, analyze, and manipulate our genetic codes as well as the proteins and, ultimately, the cells and organs that underlay human biology and extend to the universe surrounding us. These findings have led to numerous groundbreaking discoveries and the expanding potential to understand, detect, and treat diseases. We are also increasingly seeing biological systems used outside of the life sciences in a wide range of fields.
In recent years, the discussion increasingly has centered on synthetic biology, or SynBio, a term coined in the 1980s when scientist Barbara Hobom used it to describe bacteria that had been genetically engineered using recombinant DNA technology. The term has evolved significantly since then to be used more broadly and sometimes controversially.
While SynBio has already increased our understanding of biological systems and led to the availability of new tools for their manipulation and diagnostics, more recent advances in computing power and development of tools such as artificial intelligence (AI) and machine learning (ML) are leading to a further rapid acceleration in innovation. The impacts have the potential to be disruptive across many industry sectors — not just healthcare and life sciences and food and agriculture, but also industrial, chemicals, manufacturing, consumer goods, energy, and IT.
With this increasing impact across multiple disciplines and ecosystems comes an expanding need for communication and critical discourse around the implications of SynBio for society. While SynBio offers the potential to generate foods like milk or meat without animals and their associated environmental impact, we require better regulation to control quality and safety and need to understand the global and economic implications in this field. Recent examples such as the HeLa cell case and the debates around golden rice or what we are allowed to call “milk” show that we need to better address some basic questions: Who owns a gene or a cell? What level and speed of change are we willing to accept as a society? What are the risks of these new technologies? While gene and cell therapy offer the opportunity to cure serious and debilitating diseases such as muscular dystrophy or hemophilia, innovations are already enabling, for example, the synthetic creation of human embryos. What implications do these have for our future development?
Based on extensive Arthur D. Little research supported by surveys and interviews with 28 global experts in SynBio technology and applications, this Report aims to provide a comprehensive overview of the current status and prospects for SynBio, including what it comprises, the present state of technological developments, the existing development market and key player landscape, current and prospective applications, and how businesses should prepare for the future. Overall, we conclude that:
-
SynBio is an extremely diverse field. SynBio is unique in the breadth of its potential applications — from healthcare to food, manufacturing, industrial processing, and even the digital industries. The maturity of its technologies range from the embryonic to the fully commercialized. In considering its prospects, it is important to take a sufficiently granular approach.
-
SynBio technologies could have massive transformational impact in a 20-year time frame. Technologies like predictive genetic engineering, DNA synthesis for complex organisms, DNA-based storage, and AI-powered applications could transform our industries, our ability to engineer living systems, and how we cope with climate change.
-
Many SynBio applications are poised for growth. Forecast growth rates for SynBio are >20% CAGR to 2030 overall, especially in healthcare and life sciences and food and agriculture. Drivers for growth are strong, both in terms of technology push (increasing investment, lower technology costs, growing ecosystems) and market pull (especially sustainability, healthcare needs, and manufacturing efficiency). The current market is predicted to grow from around US $15 billion in 2023 to $70 billion by 2030. Perhaps more importantly, if currently developing SynBio technologies mature successfully, SynBio could have a huge impact, disrupting sectors comprising as much as 15%-20% of the entire global output in several areas in the coming decades, including:
-
Healthcare and life sciences. A large range of application areas enable personalized and potentially curative treatments as well as improvements in diagnostics and drug design and development. In the future, we expect more sophisticated treatments, greater protection from more diseases, and new applications for degenerative and genetic conditions.
-
Food and agriculture. Applications are extensive, ranging from crop domestication to synthetic food and bioremediation, with further developments in all these areas expected in the coming years.
-
Industrial and consumer goods. Applications include novel/specialty products, raw material replacements, sustainability, and process performance improvement.
-
Energy. Applications include biofuels, bioelectricity, bio-batteries, remediation, and process improvement for traditional fuels.
-
IT and tech sector. DNA-based data storage is the most promising research area but is still only at the proof-of-concept (PoC) stage.
-
-
Challenges and barriers remain high with several critical uncertainties. In many SynBio applications, development has been ongoing for decades, often driven more by technology push than market pull. Critical uncertainties include skill/competency gaps, achieving standardization of toolsets and systematization of the knowledge base, adverse public perception, ethical/biosecurity issues, availability of funding and investment, difficulties in scaling up to production levels, and poor economics compared to conventional technologies, which could hinder market growth.
-
Companies should ensure a suitable SynBio strategy is in place. Given strong drivers, accelerating technology developments, and potentially huge impacts, companies should be prepared for exponential growth that could result from breakthroughs. Given its diversity, companies need to consider carefully which fields are relevant for their business and conduct realistic market-based assessments. Key steps include:
-
Identify potential opportunities. Pinpoint those areas of the business that could be disrupted, or new opportunities created, by current or envisaged future SynBio technologies. Consider how SynBio could enhance current performance, enable new products or processes, enhance sustainability, and/or improve efficiency or customization.
-
Assess attractiveness and develop strategy. Take a market-led approach to assessing potential business cases and develop a scenario-based strategy, considering the barriers to be overcome and the critical uncertainties that remain.
-
Develop capabilities. Understand where and how to bring together the required capabilities within the ecosystem. This is especially important given SynBio’s multidisciplinary nature and shortages of specialist skills.
-
Maintain ecosystem presence. It is vital to engage in regulatory and ethical discourse and respond rapidly if and when applications become exponential.
Preamble
I am a fan of technology. Yet at the same time, I am extremely concerned about its negative externalities. Negative externalities on our children, on society, on our institutions, or on our businesses.
This concern is not new. In fact, I particularly remember reading Brave New World by Aldous Huxley in high school as a teenager. In this novel, Huxley presented a seemingly wonderful world where a mix of totalitarianism and technology resulted in an extremely stable global society without war or conflicts. By all appearances, it was the best of all possible worlds. However, individual freedoms and free will almost completely disappeared. That was my introduction to technological ambivalence.
While preparing this Report on synthetic biology with the Blue Shift team, Huxley’s Brave New World came back to me like a genetically modified “madeleine de Proust,” and I could not help but think about all the dystopian scenarios. Why? Because SynBio encompasses a multitude of potential threats and negative impacts that could arise from its misuse or unintended consequences: deadly pathogens, gene drive (which can be used to propagate a particular suite of genes throughout a population) technology, environmental and health risks, or the DIY biology movement (a movement of citizen scientists interested in SynBio experiments, which raises concerns about the misuse of easily accessible and low-cost technologies like CRISPR [defined as clustered regularly interspaced short palindromic repeat] by individuals with little prior knowledge of the field). And the list goes on!
But at the same time, I am totally amazed by the fact that most any industrial sector could leverage SynBio — not just life sciences. In this Report, we focus primarily on the positives SynBio will bring to business, society, and humans.
Before diving into it, I would like to share with you another very intriguing anagram:
“Synthetic biology”
transforms into
“hit by Scientology”
As always, anagrams move in mysterious ways.
– Albert Meige, PhD
1
What is synthetic biology & why is it important?
Mankind has long questioned the nature of life, endlessly exploring the topic through religion, philosophy, art, and science. Since the mid-20th century and the discovery of DNA, the field of SynBio has provided insights into some of the “big questions” (see Figure 1).
For example, SynBio has shown that we can synthetically create new and different functions in living organisms and harness living cells to perform nonbiological tasks. In 2023, researchers at the Weizmann Institute of Science, Israel, claimed to have grown an entity that closely resembles a 14-day human embryo, without using sperm, eggs, or a womb.[1]
Defining SynBio
Today, definitions of SynBio vary but most center on the basic concept of engineering biological systems for useful purposes. Examples include:
-
“The design and construction of new biological parts, devices, and systems, and the redesign of existing, natural biological systems for useful purposes” (Nature)
-
“A field of science that involves redesigning organisms for useful purposes by engineering them to have new abilities” (US National Human Genome Research Institute)
-
“The use of modern technologies and computation to engineer biology to solve problems related to the environment and human health” (University of California San Diego [UCSD] Synthetic Biology Institute, USA)
-
“A multidisciplinary field of science that focuses on living systems and organisms, and it applies engineering principles to develop new biological parts, devices, and systems or to redesign existing systems found in nature” (Wikipedia)
A 2023 Blue Shift global expert survey[2] carried out as part of this Report explored what distinguishes SynBio from related fields. It found that experts commonly identify convergence, engineering principles, and systems thinking as key features (see Figure 2).
While opinions broadly concur with these three aspects, experts do not fully agree on whether the source materials must be living organisms or if they can be created synthetically. For the purposes of this study and its ultimate focus on applications and impacts, we concluded that it is more useful to define the field in terms of what it produces and how it works, rather than the nature of its raw materials.
We therefore propose a practical definition of SynBio that embodies the following key features: “Engineering, modifying, or creating novel living or hybrid[3] biosystems for useful purposes.”
Supporting this definition, SynBio involves three features:
-
The convergence of multiple disciplines
-
Bridging life and physical sciences
-
At the boundary between naturally occurring and artificial worlds
-
Living or hybrid systems
-
Biomimetic engineering approaches can also be part of the SynBio toolbox, although mimicking biological systems is not a SynBio aim, per se
-
-
Applied engineering principles
-
Intentional engineering of living systems to achieve specific goals and functionalities
-
-
Systems thinking and modularity
-
Decomposition of biological systems into interchangeable modules
-
Understanding how individual parts behave and interact within the overall system
-
The spectrum of SynBio applications
SynBio covers a wide range of potential applications (see Figure 3), characterized by their degree of novelty, from more basic enhancement of existing capabilities to the complete creation of entirely new living systems.
At the next level of detail, it is useful to split the functionalities of SynBio into categories across three broad aims (see Figure 4).
As Figure 4 illustrates, SynBio can be used for making completely new products (biological and nonbiological), enhancing the performance of existing products, and improving production and manufacturing processes for the same products. In the latter category, improved sustainability is an important benefit, although better efficiency and precision are also key.
What drives advances in SynBio?
Although SynBio’s origins go back to the 1950s, breakthroughs have accelerated considerably over the last 20 years, as shown in the timeline in Figure 5.
Over the last decade, SynBio progress has further accelerated due to both technology push and market demand:
-
Technology push — convergence between ongoing developments in life sciences and physical sciences (see Figure 6), including more powerful and accurate gene-editing tools
-
Market demand — the need to meet growing demands for:
-
Greater sustainability (e.g., renewable raw materials/energy)
-
Improved healthcare (e.g., faster, lower-cost drug development; improved diagnostics; more targeted therapies)
-
Greater food security (e.g., improving yields at time of climate change and population growth)
-
Innovation (e.g., more tailored products, transformative advances in computing, and other radical new applications)
-
This combination is driving increasing public and private investment and partnerships across SynBio ecosystems. Examples within the US include:
- US government SynBio research funding increased to $161 million in 2022, with public funding totaling $820 million over the last five years.
-
The US private sector invested $8 billion in SynBio in 2022.
-
Institutions including Northwestern University, USA, and the Massachusetts Institute of Technology (MIT), USA, have received grant awards for SynBio research projects involving interdisciplinary teams.
-
New government-sponsored collaborations have launched, such as the SynBio partnership involving Ginkgo Bioworks, Geno, and Antheia.
-
New consortia have formed to develop cross-platform solutions. For example, Microsoft, Lenovo, and Twist Bioscience are collaborating to explore cross-platform work, such as through the potential of DNA data storage.
Increased activity is fueling predicted growth of the global SynBio market of 24% CAGR to over $70 billion in 2030, from around $15 billion in 2023 (see Figure 7). This covers just the provision of services, tools, know-how, and products to enable the application of SynBio by end users in markets such as healthcare, food, and industry. It does not include any potential incremental revenue these end users may realize using SynBio tools, the impacts of which we outline in the final part of this section and in Chapter 2.
Challenges to SynBio growth
Despite demonstrating impressive growth potential, SynBio development faces large-scale obstacles. These are wide ranging, from biosecurity/ethical issues to technical, economic, and consumer challenges.
Biosecurity & ethical issues
Altering biological organisms — or creating completely new ones — raises biosecurity challenges around potential unintended environmental consequences, or the deliberate misuse of SynBio by bad actors. It also leads to an ethical debate around what is deemed acceptable when it comes to changing or creating forms of life, and a blurring of the boundaries between “animate” organisms and “inanimate” machines, as detailed in Figure 8.
Global regulation is central to both ensuring biosecurity and providing reassurance around ethical concerns, such as by adopting and enforcing key principles and codes of conduct with researchers, companies, and countries.
However, regulations and guidelines are still evolving. Some overall principles have been defined, such as the long-established and internationally recognized Convention on Biological Diversity (CBD). This includes provisions related to SynBio, for example, around ensuring the safe handling, transfer, and use of living modified organisms (LMOs) produced using SynBio techniques.
However, these principles have yet to be consistently applied at a global regulatory level. Currently, fragmented national and regional regulatory frameworks equates to limited guidance over a coherent approach to the responsible use of SynBio.
Guidelines are also being developed around ethical issues. For example, codes of conduct, such as those set up for scientific research, have been created by organizations like the International Society for Systems Biology (ISSB) and the Synthetic Biology Leadership Council (SBLC). Bodies such as the World Health Organization (WHO) are forming similar guidelines.
However, along with the pace of emerging technologies, regulations are changing rapidly as well. This means that understanding and navigating different biosecurity and ethical issues remains a challenge for SynBio players.
Technical, economic & consumer challenges
At the same time, there remain significant technical, economic, and consumer acceptance barriers when it comes to accelerating the use of SynBio in many areas. These include the difficulties of scaling up processes from the lab to full production, poor cost-competitiveness with existing alternatives, a lack of sufficiently powerful technology, a need for large-scale investment, and potential consumer pushback at SynBio-derived products viewed as dangerous or threatening in some way (see Figure 9).
The transformative potential of SynBio
While it currently faces significant challenges, SynBio’s potential impact on end-user industries is vast. SynBio applications have the power to fundamentally change some of the largest industry sectors across the globe, helping solve critical challenges that threaten humanity’s future, such as those around sustainability, health, and food supply.
These industry sectors, including agriculture, healthcare, manufacturing, and data processing, represent about 15%-20% of the current global output, demonstrating SynBio’s potential significance if it can overcome the challenges and uncertainties outlined in the section above. Taking the example of the US, these disrupted sectors make up around 20% of current GDP, covering approximately $5 trillion, as illustrated in Figure 10.
Given this far-reaching and diverse potential, players in the sectors concerned cannot afford to ignore the ongoing progress of SynBio development — especially in view of the increasing strength of the drivers on both the technology and market sides.
2
The scientific & technical landscape
Although boundaries can blur, the development landscape for SynBio can broadly be split into three groups (see Figure 11):
-
Product developers — creating customer products and applications that leverage SynBio know-how and toolsets
-
Tool & technology developers — developing tools and technologies that enable SynBio products and applications, including techniques, hardware, software, and systems
-
Underlying academic research — creating basic and applied understanding of the science underpinning SynBio
As with all models, the distinctions are not always clear. For example, some tool and technology players, such as Asimov and Arzeda, are also extensively involved in product development, often in partnership with product-based businesses.
This section of the Report focuses on the academic research and tool/technology development part of the stack. We cover product developers and applications in more depth in Chapter 3.
Underlying academic research
Academic research in SynBio is extensive and covers a wide range of domains, reflecting its multidisciplinary nature. US and European institutions currently lead in investments in SynBio research, although investment in Asia is on the rise. Select examples of major academic players and their main areas of research include the following (illustrative only, non-exhaustive).
US players
MIT Department of Biological Engineering
Focusing on a wide range of relevant research for medical and life sciences applications, MIT’s centers include:
-
The Center for Multi-Cellular Engineered Living Systems — a multidisciplinary group studying purpose-driven living systems with multiple interacting living components
-
The Center for Biomedical Innovation — develops new approaches to biomedical technology, such as the structure and function of biomembrane proteins for applications in sensors
-
The Center for Neurobiological Engineering — focuses on new tools for experimental investigation of the nervous system and engineering neurons, neural tissue, and their interactions with cells, devices, and prosthetics
Northwestern University: Center for Synthetic Biology
The Center’s research is based around four themes:
-
Cell-free systems — biochemical engineering with cell-free biology activating basic cellular processes (e.g., translation) without live intact cells
-
Mammalian systems — developing novel platforms and therapeutic strategies based on custom-programming novel cellular functions
-
Enabling technologies — high-throughput experimentation tools that will allow the optimization and understanding of multi-component biosynthetic transformations, including work on engineering platforms, parallel expression of proteins, and computational analysis to identify design rules in the data
-
Ethics and societal impact — technology gaps in low- and middle-income countries, exploring the new bioethical questions that arise from SynBio development and developing ethical training for scientists
Stanford University: Synthetic Biology & Bioengineering
These two programs explore broad-based SynBio research, including protein engineering, microbiome studies, systems biology, bioinformatics, metabolic engineering, neuroengineering, biomechanics, medical devices, medical imaging, molecular engineering, microscopy, biomaterials, tissue engineering, omics/genomics, gene therapy, microfluidics, agrobiology and plant health, frugal science, and bio policy.
European players
Switzerland: ETH Zurich
The SynBio group focuses on developing and applying the tools and methods of biological computing and synthetic biology in the mammalian context, based on three pillars:
-
Fundamental, enabling technologies for precise manipulation and reprogramming of mammalian cells
-
Applying these tools to unmet needs in biotechnology and translational research
-
Development of gene and cell therapies powered by biological computing
UK: Imperial College Centre for Synthetic Biology
Research focuses on novel methods to accelerate the design-build-test-learn cycle as well as multi/interdisciplinary research, including:
-
Computational modeling and ML approaches
-
Automated platform development and genetic circuit engineering
-
Multi-cellular and multi-organismal interactions (including gene drive and genome engineering, metabolic engineering, in vitro/cell-free SynBio, engineered phages, and directed evolution)
-
Biomimetics, biomaterials, and biological engineering
The College also hosts SynbiCITE, the UK’s innovation and knowledge center, created to accelerate and promote the commercial exploitation of SynBio research and engineering biology applications. This includes the London Biofoundry, aimed at designing, engineering, and functional characterization of synthetic DNA.
Germany: Max Planck Research Network in Synthetic Biology
The network comprises 20 research groups from nine Max Planck Institutes. Research clusters include enabling technologies, life-mimicking processes, and systems perspectives. Topics covered include:
-
Engineering of genetic systems for bottom-up SynBio
-
Design and implementation of microfluidic tools for bottom-up SynBio
-
Dynamic protocellular systems
-
Recombinant protein production via cell free expression
Asian players
China: Shenzhen Institute of Synthetic Biology
Established in 2019 as the first institute of SynBio in China, the institute claims to have the world’s largest multidisciplinary team specialized in SynBio.
Singapore: Synthetic Biology for Clinical and Technological Innovation (SynCTI)
Established in 2014 as the focal research program for SynBio at the National University of Singapore (NUS), SynCTI operates as an interdisciplinary research core, interacting with other research programs within NUS and leveraging existing research capabilities in SynBio that have been developed through funding from local and international organizations.
Korea: Korea Advanced Institute of Science and Technology Department of Biological Sciences
The department declares a focus on genomics and nanotechnology, with an emphasis on research on human diseases and their molecular mechanisms, functional genomics and its application, and nano-biotechnologies and their application.
Some key research themes
Looking across the overall scientific landscape, several current SynBio research themes are expected to mature within five to 20 years with the potential for major impact to business and society. Examples include:
-
Wide-scale adoption of cell-free biomanufacturing (high impact, low uncertainty, mature within 5-10 years). The use of cell-free systems for biomanufacturing has the potential to become the new standard in SynBio. Further evolution requires overcoming the challenges of product creation beyond lab scale. Possible applications include the industrial production of green chemicals and novel products across biofuels, cosmetics, and pharmaceuticals.
-
Convergence of AI, SynBio, and automation (high impact, low uncertainty, mature within 5-10 years). Within SynBio, AI offers potential in data mining, bioinformatics, and computational biology, such as for pattern and structure recognition in gene expression data, engineering of biological systems, metabolic engineering, experiment automation, self-driving labs, outcome prediction, and reverse engineering. It offers the potential for step changes in workflow speed, accuracy, and effectiveness.
-
DNA synthesis at genome scale for eukaryotic organisms (high impact, low uncertainty, mature within 5-10 years). New technologies with the ability to synthesize and produce large-scale, complex DNA sequences are under development, including molecular assembly and cloning methods, template-independent enzymatic synthesis, microarray, and rolling circle amplification techniques. Being able to create large DNA sequences at scale and low cost would have major impact on fields as varied as sustainable materials, data storage, drug delivery, and genome engineering to generate organisms with useful properties, such as heat-resistant plants.
-
Fungal SynBio (high impact, medium uncertainty, mature within 5-20 years). Advances in applying biotechnology to mycology and fungal genetics, with advances in long read sequencing, makes it possible to generate high-quality fungal genome assemblies. Fungi have potential in a wide range of applications, such as within environmental remediation, fungal-derived food products, enzyme and other chemical production, sustainable biomaterials, fungal batteries, and many more.
-
3D bioprinting of complex animal and human tissue and organs (high impact, low uncertainty, mature within 10-20 years). 3D-printing technologies can be deployed to assemble multiple cell types, growth factors, and biomaterials. While tissue reconstruction is already feasible, reconstruction of complex organs still has many challenges. For example, heart functionalities like vascularization, mechanical load, and electrical signal propagation must be replicated. If successful, the technology offers the potential for bioartificial organ transplants as well as other medical interventions and treatments.
-
Predictive genetic engineering for multicellular organisms (high impact, high uncertainty, mature within 10-20 years). Despite progress at bacterial and mammalian cell levels, programming of higher organisms is highly complex, requiring, for example, the delivery of gene editing cascades or the engineering of whole embryos. The ability to change the physiology of animals such as worms would open possibilities and applications across agriculture, biomedical, and the environmental domain, notwithstanding potential ethical issues.
-
DNA-based data storage (high impact, medium uncertainty, mature within 10-20 years). Low writing/reading speeds and high costs per byte stored are key challenges for DNA-based data storage technologies. Current research includes using DNA nanostructures (as opposed to DNA sequences) and combinations of nanomaterials and biomolecules. Research has the potential to provide high capacity, long lifespan, and lower energy storage to meet rapidly growing data storage needs.
Tool & technology developers
Tool and technology development can be categorized usefully in terms of the design-build-analyze-enable workflow, essentially a common development engine that is fundamental to all SynBio implementation. The landscape includes large, established players; start-ups; and academia. Figure 12 provides a summary of the main tools and technologies currently in use or under development split across four categories:
-
Design — help model and simulate biological systems
-
Build — assist in construct biological systems
-
Analyze — help test and evaluate biological systems
-
Enable — accelerate the engineering process and, importantly, enable scale-up from lab to industrial production levels
The workflow is similar to that used in conventional non-bio technology development (design, build, test, manufacture), although there are differences. For example, the relative unpredictability of some SynBio processes, and the challenges of scaling up, mean that tools used to analyze and enable are perhaps even more critical.
Tool & technology maturity
Tools have varying maturity levels:
-
Design — generally the least mature (see Figure 13). While core gene and protein design and simulation tools have reached high maturity with well-established key players, the full power of AI and digital technologies remains untapped and will struggle to match demand for mature solutions.
-
Build — generally more mature (see Figure 14). Core technologies such as de novo DNA synthesis and DNA/gene delivery systems are already largely commercialized, while genome-engineering tools (e.g., CRISPR) are still maturing but have high disruptive potential and are on the cusp of wider adoption.
-
Analyze — generally the most mature (see Figure 15). Dominated by a few large players (e.g., Illumina and Thermo Fisher), rising demand for next-generation sequencing (NGS) has helped drive adoption, with the market continuing to grow rapidly.
-
Enable — varying maturity (see Figure 16). Automation and fermentation technologies are fairly mature and established, while cloud labs and microfluidics are low in maturity, with most players being start-ups. However, cloud lab companies are creating strong partnerships with large industry players, indicating the possibility for widespread adoption and growth in the coming years.
Figure 17 shows the relative maturity of selected tools within each of the four SynBio workflow stages, based on ranking by our expert survey panel. Technology readiness levels (TRLs) indicate how close a tool is to proven, commercial adoption.
Market landscape
The majority of high-profile tool and technology players in SynBio are based in America (around half on a per-company basis). The remainder are divided approximately equally between Europe and Asia, with the rest of world currently having few market entrants. The market is split between well over 500 SynBio start-ups and a much smaller number of more established players, with SynBio tool and technology players raising $10.3 billion in 2022. While most top-funded companies are currently headquartered in the US, the most dramatic growth is expected in Asia, led by China.
Many governments have targeted investments and support for SynBio. For example, the UK launched a SynBio program more than a decade ago, while the US, China, and Australia have created their own efforts more recently.
Appendix 1 summarizes selected key players across the four tool and technology development categories.
INTERLUDE #1
Evolving aesthetics — Intersecting art & science in the language of cells
Our explorations in SynBio seek to reveal hidden processes in living matter through the manipulation of genes. We aim to transform these expressions in ways they can be experienced on a human scale.
Consider an E. coli colony growing on a plate. Gradually, it spreads and — as conditions become more demanding — it creates branches and intricate patterns. This phenomenon became the inspiration for our work Stress-o-stat (see first image in this section), which explored how genetic machinery responds to cellular stress. During dire conditions, cells produce the enzyme catalase to neutralize damaging oxygen radicals. Analyzing the genetic mechanisms behind this response made it possible for us to replace the catalase gene with one producing a fluorescent protein. Our new genetic component, embedded in a genetic circuit and inserted into bacteria, caused the emission of an iridescent light during starvation. Using a drip system to regulate food release and a spiral condenser to visualize light changes, we observed the spiral light up during dire conditions and with changing nutrients we saw a slow oscillating light. Stress-o-stat aims to capture the poetic language of “stress” in bacteria, with the luminescence telling a story of resilience and resourcefulness.
To further expand on both method and metaphor, we explored how this could be done using solid media and time-lapse photography. As an additional play on the language of stress and signaling, KatEred (see second image) employed gene-producing, glowing-red fluorescent proteins. Observing growth patterns over time, a blushing red edge glowed as the colony spread across the plate, somewhat akin to tree rings telling the story of time and change. In our micro-environment, the colony creates a living visual story of struggles.
SynBio can be a portal to see processes inside cells, but we can expand on this notation by tapping into other senses like smell. Banana Bacteria (see third image) adds an extra twist by subverting our expectations. It involved the use of a genetic program that could convert an alcohol into banana oil, changing the typical off-putting smell of E.coli into a sweet-scented wonder reminiscent of bananas. It acts as a playful obfuscation that prompts us to introspect our preconceptions.
However, genetically modified organisms are restricted typically to labs; exhibiting outside these settings involves lengthy navigation of health and safety regulations as well as ethics review. Through our work, we have paved the way for the first legal exhibition in showing genetically modified organisms in the UK and beyond its borders.
These works make invisible processes perceptible to humans. Yet, the absence of real-time interaction creates a barrier in how we experience these organisms and processes, often requiring us to add an interface like time-lapse to bridge the experience. Our initial real-time experiments used nanomagnetic particles, which allowed participants to control individual cells using an external magnet.
Surprisingly, we found that magnetic bacteria occur naturally. These organisms swim along the Earth’s magnetic field to optimize their position in a microaerophilic gradient. Exposing these bacteria to a changing magnetic field causes their bodies to reorient and, in doing so, scatter light. In liquid culture, the scattering can be observed as a visible shimmer resulting from blocking or passing light. This extraordinary phenomenon inspired our work Living Mirror (see fourth image). As a mirror, it draws on the idea of water as our original interface predating today’s screen-based technologies and references the myth of Narcissus, who tragically drowned while captivated by his reflection — a reminder of how we continue to immerse ourselves in similar mirrors as we extend our identity into the virtual. To create the mirror, we used a camera to capture a person’s image converting pixels into numerical values. This data activated individual magnetic coils, orchestrating bacteria to produce hundreds of light pulses that formed an image in real time. The work delves into the concept of “self” and how we are predominantly composed of nonhuman bacterial cells. It aims to capture a duality, both technically and conceptual, of how we navigate the intersection of digital and biological perceptions of self.
— C-LAB, an art-science collective founded by Howard Boland and Laura Cinti that combines scientific advancements in the biosciences, critical thinking, and future possibilities through hands-on science
Stress-o-stat
Stress-o-stat is a living artwork that visually captures stress in bacteria as light. The installation combines scientific instruments, methods, and aesthetics to produce a real and experimental device. SynBio tends to postulate a machine-like language onto the living, as something programmable. Paradoxically, it is the opposite of the digital; instead of the machines becoming life-like, it suggests life becoming machine-like.
KatEred
KatEred explores a living visualization of oxidative stress using a glowing-red fluorescent protein and through the use of time-lapse. The colony creates a blushing glow around its edges and creates an imprint of cellular stress over time. Red tends to signify an alert and has been used as “official” semantics in many apparatuses such as warning lights. While these factors play little in a biochemical role, they play with our perception as visual readers.
Banana Bacteria
Banana Bacteria is an olfactory work that explores the paradox of having the foul smell of bacteria exchanged with the sweet smell of banana. These bacteria have been genetically altered by removing a gene responsible for the foul smell commonly found in bacteria and adding a genetic design that enables bacteria to synthetically produce banana oil.
Living Mirror
Living Mirror is a bio-computational imaging system where magnetic bacteria scatter light under a changing magnetic field. This phenomenon was used to create a bio-computational system containing bacteria and individually controlled magnetic coils to recreate patterns and potentially real-time images of people.
3
The applications & industry landscape
SynBio underpins a diverse range of applications across many industries, led by healthcare and life sciences and food and agriculture. The application landscape demonstrates the enormous scope of SynBio. For example, it can be used to create vaccines, improve crops, generate biofuels, and enable DNA-based data storage. Consequently, there are widely varying levels of maturity among applications, ranging from early stage through to full commercialization.
Identifying SynBio opportunities
Our 2023 global expert survey explored industries that would be most impacted by SynBio in the next five to 10 years (see Figure 18). About a quarter of respondents identified healthcare and life sciences as the sector that will see the greatest applicability of SynBio, followed by food and agriculture (21%), and then industrial, manufacturing, chemicals, and materials (18%).
Application maturity
Mapping selected SynBio applications against the Gartner hype cycle further demonstrates both their range and the diversity of their maturity levels (see Figure 19):
-
Healthcare and life sciences. Various subsectors in healthcare are at differing points on the curve. For example, widely used monoclonal antibodies as a treatment option are at the peak of productivity, whereas newer therapeutic modalities like gene therapies are considered to be at the peak of inflated expectations.
-
Food and agriculture. This sector is approaching the peak of inflated expectations, as it is maturing with products that are commercially available, yet market uptake is still relatively limited. Newer technologies that use SynBio techniques are on the brink of commercialization and those that address current sustainability challenges around food production (e.g., cell agriculture for cultured meat) are at the technology trigger stage.
-
Industrial, manufacturing, chemicals, and materials. This segment is approaching the peak of inflated expectations, as demand for novel enzymes for many end-use industries continues to grow. Commodity chemicals are also at the peak of an inflated expectations phase, while partnerships between industry players (e.g., Unilever) and SynBio companies continue to form.
-
Consumer products. Approaching the slope of enlightenment, this segment is maturing with some commercially available products (e.g., fragrances, plant-based leather alternatives). With sustainability at the forefront of consumers’ minds, the use of SynBio to generate consumer products will continue to grow.
-
Energy. This sector is at the trough of disillusionment, as biofuels and bio batteries continue to be slow to overcome economic and technical barriers. However, next-generation biofuels produced from waste materials and other co-products will still have applications in, for example, heavy-duty transport, such as aviation and shipping, that are difficult or impossible to electrify.
-
IT and technology. Just beyond the technology trigger phase, major players are starting to investigate the adoption of SynBio tools and techniques like DNA data storage. This is evident through the growing number of partnerships between SynBio companies and tech players (e.g., Twist and Microsoft; Illumina and Dell).
Key areas for growth
Comparing growth and maturity provides an indication of the significance of each of the key application sectors today (see Figure 20).
As Figure 20 illustrates, healthcare and life sciences is the most mature and largest market, with one of the highest growth rates. Food and agriculture is next, although there is still extensive tech development in this segment at low TRLs. Industrial/manufacturing/chemical/materials, consumer goods, and energy are also growing, although barriers toward commercialization are higher, with IT and tech being the most embryonic. However, if DNA-based storage could be commercialized, this could become a huge market.
The remainder of this chapter takes an in-depth look at each of these markets, including potential applications, opportunities, and challenges.
Healthcare & life sciences
Motivations for SynBio applications in the healthcare and life sciences sector are strong. Key catalysts include:
-
Rising incidence of chronic and debilitating disorders (e.g., cancer). The aging population and resulting rise in chronic diseases increases the need for more specialized treatments.
-
Shift toward personalized/precision medicine. The movement toward more targeted, individualized treatments/therapeutics will increase SynBio use, as it enables fine-tuning of organisms to perform specific/dynamic functions.
-
Limitations of current treatments. SynBio can improve both efficacy and safety of current treatments, as well as satisfy the need for a new treatment method and mechanism.
However, success will require the sector to overcome existing challenges in four key areas:
-
Regulations. Strict regulations regarding drug development and a relatively untested regulatory environment make the process lengthy and costly.
-
Ethical limitations. Public hesitancy and debates regarding ethics of modifying the genome hinder SynBio expansion.
-
Limited technological capacity (e.g., data streams and computer capacity). Technological capacity limitations hamper the ability to actualize all possible SynBio developments.
-
Complexity of large-scale production and manufacturing of cell therapies. The complexity around manufacturing cell therapies and the limitations of large-scale production complicate the process of taking a SynBio concept from lab to commercial scale.
Application areas (non-exhaustive)
Tissue engineering
SynBio enables the creation of multicellular structures for regenerative medicine, drug testing, and beyond, including tissues and organs using cells and structural components to mimic the morphology and function of native tissues.
For example, gene therapy, advanced biologics, and small molecules are being studied to stimulate the regeneration of damaged heart cells. SynBio can be used to generate tissues that can then be transplanted. As an example, cultured epidermal autografts are being used as a permanent skin replacement for the treatment of patients with serous burn injuries.
The tissue engineering market is already large and well established at around $15 billion globally in 2022, with growth of more than 15% CAGR to 2030. Most SynBio applications are still at foundational stages, with key players like Integra LifeSciences and C.R. Bard (acquired by BD in 2017) still focusing mainly on conventional technologies. Start-ups such as Organovo focus on new 3D biomaterial printing.
Medical devices & implants
Integrating SynBio into wearables could expand opportunities for noninvasive monitoring of physiological status, disease states, and exposure to pathogens or toxins to practically enable biosensors. However, sustaining living organisms in medical devices for extended periods remains a challenge.
Potential application examples include new wearable or implanted medical devices for health monitoring and control. Demonstrating this trend, a prototype face mask with a lyophilized (i.e., freeze-dried) CRISPR sensor has been developed for wearable noninvasive detection of SARS-CoV-2 at room temperature.
Vaccines
Vaccines are commonly prepared from an inactivated or weakened form of the causative agent or from its constituents. SynBio facilitates shorter development cycles through alternative technologies, such as nucleic acid vaccines (e.g., mRNA), viral vector vaccines, or recombinant vaccines (e.g., SHINGRIX).
For example, synthetic RNA platforms allow for rapid, scalable, and cell-free manufacturing of prophylactic and therapeutic vaccines. The Pfizer-BioNTech COVID-19 RNA vaccine was the first to be approved for humans. Moderna and BioNTech are examples of key players that used synthetic messenger RNA to protect against COVID-19.
Stem cell therapies
Stem cells serve as the raw material from which all specialized cells are derived, capable of generating healthy replacements for cells impacted by disease (regenerative medicine). Achieving this transformative process is made possible through genetic reprogramming techniques such as CRISPR. For example, stem cells can be manipulated into heart muscle cells, which can then be injected into the heart muscle of a patient with heart disease. The transplanted cells would then aid in repairing the injured muscle.
The global stem cell therapy market was valued at ~$10 billion to $12 billion in 2022 and is predicted to grow at a 10%-15% CAGR for the next 10 years. An influx of interest and funding has resulted in both emerging players, like bluebird bio and BlueRock Therapeutics, and more established players, like Mesoblast and Osiris Therapeutics, coexisting in the expanding market.
Cell therapies
SynBio enables the development of therapies based on bioengineered cells. In these currently highly personalized treatments, cells are becoming the treatment modality. Among the first treatments in this space was Kite Pharma’s CAR T-cell therapy, but there are many more in development to treat a variety of disorders and diseases. For example, CAR T-cells have successfully treated blood tumors such as large B-cell lymphoma and also promise a new scenario of therapeutic interventions for solid tumors.
The genetically modified cell therapy market is projected to grow rapidly from $2.7 billion in 2022 to $32.3 billion in 2029 at a CAGR of 42%. Main players in the space include large pharma players such as Bristol Myers Squibb and Gilead, which have expanded their cell therapy capabilities through acquisitions of Juno/Celgene and Kite Pharma, respectively. However, there are several smaller biotech players harnessing this technology with assets in the early development phases, including Allogene and Ixaka (formerly Rexgenero).
Gene therapies
SynBio has enabled scientists to cure diseases by correcting the underlying genetic defects through genomic editing (e.g., CRISPR) or introduction through a vector (usually a viral vector). Alternatively, synthetic oligonucleotides can be used to modify the genetic response, with RNAi currently being the most prominent technology. For example, vector-based gene therapies have been approved for both hemophilia A and B. In both cases, viral vectors are used to deliver the correct gene and potentially cure the patients through a single treatment, although long-term data is not yet available.
The gene therapy market is projected to grow rapidly at a CAGR of ~20% from $7.5 billion in 2022 to ~$30 billion in 2030. Examples of key established players are large pharma players such as Novartis as well as more niche players like Vertex. The approved gene therapies are currently centered on hematological genetic diseases, such as hemophilia and severe sickle cell anemia, but also include degenerative inherited diseases. Gene therapies for some larger indication areas, such as type 1 diabetes, are also in development.
Bio-machine interfaces
SynBio technology could provide solutions for developing effective bio-human-machine and neuro-machine interfaces. For example, it has been demonstrated that closed-loop brain-machine interface (BMI) technology can modulate sensory-affective experiences in rats, illustrating how a BMI approach could be used for pain control as an alternative to drugs. Numerous healthcare applications exist, such as restoring physical functions and controlling symptoms. There are also applications in education and entertainment, where BMI technology could be used, for example, to control avatars and objects, contributing to a more immersive gaming experience.
Maturity timeline
In the future, SynBio is expected to lead to more sophisticated treatments, greater safety from more diseases, and new applications for existing conditions, such as neurological and genetic ailments. Figure 21 outlines the maturity timeline across the healthcare and life sciences sector.
Food & agriculture
Feeding a growing global population while coping with the immediate impacts of climate change is a critical challenge for the world for several reasons:
-
Sustainability and accompanying policy and regulatory changes. Demand is growing for SynBio solutions due to their ability to provide results while protecting the environment and maintaining or improving soil fertility.
-
Population growth. Population growth has been projected to require improvements in productivity/yield by 60%-80% up to 2050, increasing demand for SynBio improvements. Initiatives are increasingly being driven by governments (e.g., Singapore’s 30 by 30 food security and sustainability plan).
-
Plateauing yields with current improvements. Limitations in current farming improvements, such as fertilizers and greenhouses, are making the industry look to other more effective options for higher yields.
Successful SynBio adoption will require the sector to overcome existing challenges in three key areas:
-
Regulation. Elements of the emerging regulatory environment remain untested, creating compliance challenges.
-
Public perception. Consumers, especially in Europe, have negative views of existing genetically modified food and technologies (e.g., gene/genome editing). This negatively impacts demand for SynBio-derived products and potential market growth.
-
Risk aversion. SynBio adoption involves disruptive change within the market. Larger companies with established systems, in particular, are reluctant to change how they operate, holding back adoption.
Application areas (non-exhaustive)
Crop domestication & breeding
SynBio enables metabolic pathways, genetic circuits, and plant architectures to be engineered for agricultural plants, enhancing their suitability to human needs, such as around taste/flavor, color, yield, storage, productivity, amino acid production, ability to fix nitrogen, and disease resistance. SynBio offers a major enabler to reduce the time required for crop breeding cycles by making use of existing genomic data sets, as well as deploying other toolsets to expedite the breeding cycle which, for some crop varieties, may take five to seven years. For example, Pivot Bio used an engineered bacterium to create a biological nitrogen fertilizer for corn, reducing the need for chemical fertilizer while increasing yield.
The crop-breeding market is expected to reach over $30 billion by 2029. Major players, such as Benson Hill and Apeel, are being joined by smaller companies like Tropic Biosciences.
Precision agriculture
SynBio can improve the physical properties of soil in a variety of ways; for example, through improving soil microbiomes via microbial fertilizers, enabling soil remediation, and developing natural pesticides, as well as helping to diagnose potential pests and diseases in soil and water. This type of improvement includes the use of novel bio-fungicidal seed treatments to control soil-borne fungal pathogens that cause seed rot, damping-off, root rot, and seedling blights while minimizing environmental impact.
The global precision agriculture market reached a value of around $6.7 billion in 2021. Governments across the globe have undertaken several initiatives to encourage the uptake of modern agricultural techniques to enhance crop yield and productivity. Syngenta Group and Bayer Crop Science are leaders in precision agriculture. However, as the need for precision agriculture continues to grow, there are several start-ups and smaller players entering this space, such as Pivot Bio, Concentric, and Robigo.
Designer synthetic & bio-based food
SynBio can be applied to engineer food not produced in natural soil or obtained from animals (e.g., microbial nutritional protein). Such food is produced artificially in both laboratory and industrial conditions, using a culture or growth medium. For example, in 2016, Impossible Foods launched the Impossible Burger, a vegan alternative to a beef hamburger. The process uses genetically engineered yeast to produce the heme protein needed to make the food look and taste more like meat.
The global synthetic food and ingredients market is estimated at around $12 billion to $13 billion in 2022. Impossible Foods and Beyond Meat currently dominate the plant-based meat market. The increasing number of new plant-based food start-ups and the expansion of the food service industry will further drive growth. Start-ups providing animal-free dairy proteins, including ImaginDairy and Perfect Day, are also rising in popularity.
Bioremediation, biosequestration, biosensors
SynBio can be used to create genetic-engineered microorganisms and microbial consortia to improve the efficiency of bioremediation, especially in heavily contaminated or difficult-to-access environments. An example of this is the work of UK-based CustoMem, which uses SynBio to create a granular material that attracts and sticks to micropollutants, such as pesticides, pharmaceuticals, and certain chemicals in wastewater.
The global bioremediation market is estimated at around $13 billion to $14 billion in 2022. Growth will be driven by increasing environmental awareness as well as regulatory demands, with bio alternatives perceived as more natural, as well as being cost-effective in many cases. The market is fairly fragmented, but major players include Xylem, Drylet, Aquatech, and others.
Maturity timeline
Moving forward, we expect SynBio adoption to lead to further developments in crop technologies, food engineering, and environmental remediation approaches across the food and agriculture sector (see Figure 22).
Industrial, manufacturing, chemicals & materials
The need to decarbonize, reduce waste, and become more sustainable is increasingly impacting industrial companies and their operations. Key drivers include:
-
Sustainability needs and accompanying policy changes. Increased global awareness of environmental issues will likely result in further regulations restricting fossil fuel manufacture.
-
Technological advances enabling new innovations. New and evolving technology will reduce the cost and complexity of applying SynBio developments.
-
Waste reduction from large-scale production. SynBio technologies can both improve and replace current manufacturing processes that produce large amounts of waste.
-
Rare earth and mined materials supply constraints and security. The transition to net zero will require huge increases in mined raw materials, especially metals. This will increase further the need for more sustainable production processes.
However, major challenges to SynBio adoption remain in three key areas:
-
Cost. Though more sustainable, the high cost and greater energy consumption of SynBio processes at scale are major barriers to adoption.
-
Risk aversion. Players with large-scale, established infrastructures (e.g., chemicals industry) and profitable systems are hesitant to adopt SynBio alternatives, due to the high costs and risks inherent in such a major transformation.
-
Collaboration. Many SynBio solutions require the formation of new ecosystems, creating nontraditional partnerships; for example, between materials companies, technology providers, manufacturers, consumers, and environmental companies.
Application areas (non-exhaustive)
Bio-based specialty products
Bio-based specialty products can be created via new production pathways where microorganisms generate a wide variety of chemicals. While many processes are already established, SynBio offers the potential to improve them. For example, the hyaline family of clear, flexible films are used for flexible electronics (e.g., foldable smartphones and wearable electronics).
The bio-based specialty products sector is primarily dominated by larger players, including BASF, Toray Industries, and Novamont. These companies have significant financial backing and partnerships already in place. Companies like Toray have developed plant-based synthetic fibers, while Novamont has focused on the development of biodegradable bioplastics by applying SynBio techniques.
Sustainable production/manufacturing
Using microorganism strains engineered to produce defined outputs (e.g., production of small molecules of high value) helps reduce reliance on petrol-based and even plant-based chemicals and processes. For example, engineered microbes and biotechnological copper-recovery applications are already being applied in copper mining, including for bioleaching, accumulation, and recycling.
The market for sustainable production/manufacturing is extremely broad and diverse, including, for example, pharmaceuticals, commodity chemicals and materials, consumer goods, and bioremediation, all of which are covered elsewhere in this Report.
Commodity chemicals & materials
Commodity chemicals can now be engineered and produced from microbes and more renewable materials (e.g., value-added chemicals can be synthesized from flue off-gases). As one example, Biomason uses bioengineered bacteria to “grow” bricks. The company claims these “Biocement” bricks are as strong as traditional cement and are made on-site using local materials.
Biotech companies such as Ginkgo Bioworks and Genomatica have focused on the commodity chemicals and materials sector, with Gingko valued at $15 billion in 2021. Genomatica has developed partnerships with Unilever to develop alternatives to palm oil and fossil fuels. The company has also partnered with global chemical company Asahi Kasei to commercialize renewably sourced nylon.
Enzyme production
SynBio techniques such as DNA synthesis and heterologous expression can be used to produce novel enzymes with improved properties. For example, Arzeda has developed Intelligent Protein Design Technology, which combines physics-based protein design and AI to create new designer proteins and enzymes, which it claims to be more efficient and less risky than conventional approaches.
The rising demand for novel enzymes is attributed to growing product demand in various end-use industries (e.g., detergents for industrial applications). Established players like Novozymes and BASF have several partnerships in place with companies across consumer care and biopharmaceuticals. Additionally, there are many less mature players, including Arzeda, that have received limited funding but have developed significant partnerships with companies, including Unilever, Amyris, and BP.
Maturity timeline
In the future, we expect increased penetration of SynBio technologies into industry and manufacturing, provided that the economic challenges can be solved (see Figure 23).
Consumer goods
Sustainability and personalization, particularly in the luxury goods and fashion sectors, are strong drivers, in addition to the following:
-
Sustainability and accompanying policy changes. SynBio solutions typically offer sustainability benefits for governments looking to meet sustainability goals.
-
Consumer awareness. There is increasing consumer awareness and demand for more sustainable production and products.
-
Personalization. There is a growing market for designer, personalized, and high-end consumer products. Personalization is a major trend in luxury consumer goods.
-
Rising energy prices. High energy prices are driving manufacturers to seek alternative processes and less energy-intensive production processes, perhaps even at the expense of time-to-product.
Successful SynBio adoption will require the sector to overcome existing challenges in three key areas:
-
Scale. Many consumer products using SynBio can only be produced on a small scale. The cost and time-to-market for these products may therefore be very high, especially given that some involve relatively novel technologies.
-
Cost. The cost-effectiveness and established infrastructure for fossil fuels in commercial production can make changing to SynBio economically unattractive, particularly as it is a less mature technology.
-
Public perception. Consumers may be wary of SynBio products, particularly in areas where “natural” products are seen as premium, or where humans are in close physical contact with the product (e.g., cosmetics).
Application areas (non-exhaustive)
Flavors & fragrances
By applying biosynthesis and precision fermentation, SynBio creates alternative pathways for molecules traditionally sourced from plant or animal materials. For example, Evolva applies precision fermentation to develop flavors and fragrances, such as nootkatone, valencene, vanillin, and L-arabinose.
The global fragrances market has been valued at $59 billion in 2023. Both Amyris and Conagen are mature players in the SynBio-based fragrances space. Amyris has had several partnerships to develop bio-based fragrances, including with Maison Francis Kurkdjian. Conagen is a global leader in developing natural fragrances and its vertical integration has enabled it to rapidly commercialize fragrances.
Consumer materials, textiles, fabrics
SynBio provides the ability to create more sustainable (carbon-negative) textile materials, both improving existing textiles and creating novel, animal-free materials. For example, Hide Biotech produces a leather-like material by transforming food industry waste into a customizable collagen-based leather substitute.
The global vegan fashion market, which includes any apparel not using animal products including non-synthetic materials, was valued at around $450 billion in 2021 and is expected to grow at a CAGR of 7.1%. While there are several small players entering this space, more established players like Bolt Threads have established strong partnerships with the likes of Adidas and Stella McCartney. Genomatica has developed a novel plant-based nylon and has recently partnered with lululemon to use its sustainable fabric. Most players in this space are focused on developing alternative materials to offer consumers more sustainable choices.
Consumer care products
SynBio can be applied to develop ingredients and compounds for skincare and beauty products, replacing less sustainable production processes and moving away from animal-derived products. As an example, Biossance produces skincare products based on a skin-identical hydrating molecule, squalene, made from sustainable fermented sugarcane.
There are a several SynBio companies with commercialized products in the consumer care space. Companies like Amyris have successfully developed trans-β-Farnesene-derived squalene, which is used across several personal care products today. As another example, haircare company K18 has patented K18PEPTIDE, a high-performance ingredient that reverses hair damage at the molecular level. Since its launch, the product has seen significant consumer adoption.
Consumer healthcare & wellness
Ingredients for health and nutritional products can be made from more renewable alternatives through SynBio. For example, Ayana Bio has launched lemon balm and echinacea products made via plant cell culture, which it claims can provide a consistent supply of botanicals without the complexities of existing supply chains.
The largest player in the consumer healthcare and wellness space that leverages SynBio is Conagen, which has recently developed resveratrol made by clean bioconversion. Other players with commercialized products include ZBiotics, which offers a product that helps the gut break down acetaldehyde, and Sun Genomics, which produces custom probiotics.
Maturity timeline
Moving forward, we expect wider ranges of SynBio-produced consumer goods that offer greater personalization and tailoring (see Figure 24).
Energy
Energy players have to decarbonize to meet increasing regulations and net zero targets while ensuring energy security and coping with the impacts of geopolitical uncertainty. As such, the relevant drivers for SynBio solutions include:
-
Sustainability and accompanying policy changes. Fluctuations and potential increases in fuel prices driven by introduction of carbon taxes, scaling back of oil production, and geopolitical uncertainty could make bio-based routes more attractive.
-
Energy insecurity. This is driving a significant push to move away from depleting fossil fuels, resulting in increasing demand and willingness to pay for SynBio fuels.
-
Incremental biofuel uses. Bio-based fuels may add incremental value in extreme weather conditions or specific applications, such as aviation and marine fuels, where electrification is not a viable option.
Successful SynBio adoption will require the sector to overcome existing challenges in three key areas:
-
Cost. Biofuels are generally much more costly than fossil fuels, and there are often major raw material logistical challenges. The established systems and infrastructure of fossil fuel use, and huge upfront investments required for conversion, hinders the establishment of biofuels as cost-effective options on the market.
-
Resources. Despite innovations to date, current biofuel production technologies do not consistently deliver the volumes required for commercial production, resulting in inefficiencies in scale-up.
-
Electrification. A shift toward electricity for mobility implies an overall decline in oil use, even including bio-based alternatives. This uncertainty further hinders investment decisions.
Application areas (non-exhaustive)
Biofuels
Organisms can be engineered using SynBio to produce biofuels from waste materials or biomass feedstocks rather than food crops, as well as to produce novel fuel molecules with better performance/higher energy content than current fossil fuel alternatives. For example, the metabolic engineering of yeasts is enabling microbial cell factories for biofuel production.
The second-generation biofuels market — comprising fuels produced from waste materials rather than food crops — was valued at $6 billion in 2020 and is projected to reach $59.4 billion by 2030. Today, this comprises mostly hydrogenated waste vegetable oil produced by Neste and some long-established smaller businesses seeking to use genetically engineered microorganisms for advanced fermentation. Companies such as Poet have tried to make this technology work at scale for many years but have faced significant headwinds, mainly due to feedstock availability and pre-treatment issues.
Bioelectricity & bio-batteries
SynBio techniques can be used to create engineered organisms that produce “cell factories” capable of generating energy from organic materials. These include organisms capable of producing bioelectricity and photosynthetic biomaterials for solar panels, for example. Bio-batteries are distinct from microbial fuel cells, which produce electricity from the microbial oxidation of reduced compounds. As an example in this area, scientists at the UK’s Defence Science and Technology Laboratory are researching a bio-battery based on DNA technology that will be much safer in the case of destruction during conflict.
Microbial fuels cells have been under development for many decades, but their application has been limited due to high electrode costs and low power. Bio-batteries, which are based on the same principles as human/animal digestion, are still in their infancy. Recent examples involve battery concepts based on lactates with enzymes bound to a structural scaffold.
Bioremediation & biosequestration for energy
SynBio can be used to improve processes for waste treatment, such as by speeding up or enabling natural decomposition. These methods can be used to treat waste in situ, such as following an oil spill (especially on land), while also having the theoretical potential to convert waste to energy, though processes are extremely slow and inefficient today. For example, LanzaTech’s carbon recycling uses engineered bacteria to convert pollution to fuels and chemicals.
The global bioremediation market in 2022 is thought to be worth around $13 billion, with most forecasts suggesting growth of 9% CAGR to around $30 billion in 2030. Metabolic engineering, enzyme modification, and other SynBio technologies are key enablers. Bio-based routes to carbon sequestration through the capture of flue gases continue to be largely economically unviable, although players are making progress in this area, with LanzaTech currently leading the field.
SynBio for hydrocarbon extraction
SynBio techniques can improve the extraction of traditional hydrocarbon or fossil fuels, such as through enhanced oil recovery techniques (EOR) to help process more difficult-to-extract reserves from depleted oil wells. Today, the use of SynBio in EOR is still an emergent technology that is relatively slow and inefficient and has not yet been proven at scale. However, recent lab-scale research is showing progress. For example, a recent study published by the American Chemical Society has shown that biosynthesized nanoparticles can be used to increase oil recovery rates by several times.[4]
Maturity timeline
Moving forward, we expect to see further improvements in biofuel viability as well as other solutions, including electricity and the circular economy (see Figure 25).
IT & technology
In an increasingly digital world, mankind is producing, storing, accessing, and analyzing progressively larger amounts of data. Volumes are growing exponentially, pointing to future needs for new forms of data storage, cybersecurity, and novel computing paradigms that can solve currently intractable problems.
While still in its infancy, DNA data storage could ultimately provide much higher storage density and ease of synthesis, while biocomputation and bio-inspired cryptography offer future advantages over current methods.
In IT, SynBio applications are still at the PoC stage and must overcome at least five key challenges:
-
Immaturity. Error rates in DNA computing need to improve to allow biocomputing and cryptography.
-
Technical limitations. The capabilities within encoding, writing, storage, reading, decoding, and so on, render DNA storage currently uncompetitive with magnetic and solid-state storage devices.
-
Cost and throughput barriers. Despite theoretical advances in lower-cost synthesis techniques, commercial synthesis costs have not changed drastically, limiting large applications. Additionally, current DNA sequencing throughput means intended applications are uncompetitive.
-
Slow commercialization. There is a lack of current initiatives to turn lab research into applications accessible by end users, hindering rapid commercial adoption.
-
Competition. Extensive development is taking place using a range of other computational and data management technologies with promising results in some areas.
Application areas (non-exhaustive)
Data storage
By nature, DNA is used to store genetic information. However, it can also be used as a storage replacement for magnetic and optical media. DNA can store data for over 500 years with little to no data loss and is extremely dense, holding up to 1 exabyte per gram. Data can also potentially be stored inside living cells, opening up applications dubbed DNA of Things (DoT). For example, start-up player Catalog claims that its current Shannon prototype computation and storage platform has demonstrated PoC.
As far back as 2015, Microsoft invested in a project with the University of Washington, USA, to develop molecular-level data storage. And in 2020, Illumina, Microsoft, Twist Bioscience, and Western Digital created the DNA Data Storage Alliance to build an interoperable storage ecosystem based on DNA as a data storage medium.
Biocomputation
Biocomputers use biologically derived materials to perform computational functions. Types include biochemical, biomechanical, and bioelectronic computers. In theory, their abilities to self-replicate and self-assemble could lead to low-cost production in the future, while they may ultimately have the potential to deal with “intractable” problems that are hard to solve via conventional computers. Biocomputation is still at an embryonic stage. While examples have been created that can perform simple binary logic calculations, an engineered biocomputer is thought to be at least 20+ years away.
Today, biocomputation is driven mainly by universities, like the Centre for Biotechnology and Plant Genomics at Technical University of Madrid, Spain, and a few start-ups, such as Catalog. Additionally, companies such as Biocomputation Lab offer biocomputation services (e.g., designing biocircuits to perform predefined functions, mathematical modeling, and computational simulations to understand biological systems).
Cryptography
Bio-inspired cryptography is an emerging approach leveraging the high storage information density and parallelism of DNA. While it has been used for encryption and decryption algorithms, it is still at an embryonic stage, with no current examples of its deployment. Research institutes and universities like the University of Potsdam, Germany, are engaged in developing a molecular labeling system with position-oriented DNA encryption. Catalog’s claimed DNA-based computation device should also cover cryptography.
Maturity timeline
Full-scale DNA-based data storage systems and DoT applications are estimated to be at least 10 years away (see Figure 26).
INTERLUDE #2
Philosophical & ethical issues surrounding SynBio
SynBio has piqued the interest of philosophers and ethicists, leading to a cross-fertilization among various subfields of philosophy, including philosophy of science, branches of applied ethics, as well as philosophy of technology and areas of the social sciences that address questions about responsible research and innovation — and the broader relationship between science and society. Researchers in these domains are working to clarify relevant concepts in relation to specific areas of SynBio. Protocell research, for example, has sparked philosophical debates about the explanation and definition of living systems and the concept of emergence. Targeted mutagenesis in plants has stimulated thinking about the definition and ethical relevance of “naturalness”: Is a plant that is a result of targeted mutagenesis still a natural object, is it a technological one, or is it a kind of hybrid? For whom does it matter?
Conceptual clarification like this can help inform normative questions pertaining to the right course of action. For example, to what extent should we be concerned about our abilities to intervene intentionally in the most basic and fundamental of vital processes, often referred to as “playing God”? What is the best way to govern the complex relationship between research, government, and private interests in SynBio? How can we ensure that risks emerging from technologies that can intervene in the basic processes of life to create new functionalities or even new organisms are managed without obstructing their potential to create various forms of value?
These questions are multifaceted. The concern about “playing God,” for instance, takes two main forms: one is primarily ethical and concerns the normative limits of instrumental human control over the natural world; the second relates to whether public concerns about synthetic biologists playing God might impede progress in the field. In the first case, philosophers have pointed out that there is nothing novel about humans instrumentalizing other forms of life and trying to exert control over evolution and development (with agriculture being a primary example).
The second concern, relating to public attitudes toward science and democratic governance of science, was somewhat quashed by public consultations demonstrating that public concerns about SynBio relate more to questions pertaining to the distribution of benefits and risks: who controls these new technologies and to what end? — traditional political questions. These attitudes toward SynBio track earlier reports of public perceptions of similar technologies, such as GMOs in agricultural biotechnology.
From a sociopolitical perspective, SynBio has stimulated discussion about the governance of “technoscience,” forms of scientific activity requiring sophisticated technical infrastructure that necessitate large-scale public or private investment. In this way, SynBio has been an excellent case study for discussion on the role of democratic decision-making mechanisms in science and research governance and the implementation of responsible research and innovation in publicly funded research programs.
Overall, SynBio continues to require ethical and political guidance but also stimulates new pathways of inquiry for classical questions in philosophy, ethics, and politics.
— Darian Meacham, Maastricht University
Darian Meacham is Chair of the Philosophy Department at Maastricht University, the Netherlands. He is also the Principal Investigator for Ethics and Responsible Innovation at the Brightlands Institute for Smart Society (BISS). From 2015–2019, he worked at BrisSynBio, a multidisciplinary research center based at the University of Bristol, which focuses on the biomolecular design and engineering aspects of synthetic biology.
4
How companies should respond
A wide range of companies could see their current or future businesses impacted by SynBio, including:
-
Companies that have relevant capabilities for the current/future SynBio design-build-analyze-enable workflow
-
Companies in application sectors such as healthcare and life sciences, food and agriculture, industrial/manufacturing, consumer goods energy, or IT and technology
-
Investors who may be considering taking a position in respect of actors in the SynBio value chain
These organizations should consider four steps to assess how SynBio could impact their business (see Figure 27).
Identify potential opportunities
Identifying opportunities must be both systematic and creative. A good starting point is to understand the areas of the business that could be disrupted, or where new opportunities could be created, by current or envisaged future SynBio technologies.
For example, the taxonomy outlined earlier in Figure 4 is a guide to finding opportunities where SynBio could enable:
-
Creation of novel products or use of new/different raw materials
-
Enhanced performance of existing products, either directly or through substitution
-
Improved sustainability of existing production processes
-
Greater process efficiency, better precision, or more customization in production and manufacturing
As always, combining a systematic product/process review together with open-ended what-if exercises is likely to produce optimal results.
Assess attractiveness & develop strategy
Even with early-stage applications or those that are not yet mature, it is important to consider the potential business case. This is especially true in SynBio, which often has been characterized by a “solutions looking for a market” philosophy. It is therefore important to take a market-led, business model approach, considering the barriers that need to be overcome to move from viable product to full commercialization (see Chapter 3 for more details on these barriers).
Given the difficulties and challenges of scaling production, it is likely that high value-add-niche SynBio applications will often be more attractive than those where the business model requires high-volume production.
It is valuable for companies to outline a strategy — even if at only a high level. Ideally, the strategy should be scenario-based to reflect critical uncertainties like skills/funding/investment availability, public perception/ethics, and overcoming scale-up challenges. It should include SynBio status, objectives, priorities, programs, and a roadmap to monitor progress.
Depending on the range of opportunities identified, a portfolio approach can be valuable, as it provides a balanced program of projects to counteract the challenges and potential long-term nature before SynBio opportunities deliver results.
Develop capabilities
SynBio is a multidisciplinary field and requires many specialist capabilities. It is extremely unlikely that any one player will have all these capabilities and skills in-house, so understanding the player landscape is essential.
Once a company has selected particular opportunity areas, it should engage with relevant key players in the partner ecosystem. Understand that for many application areas the ecosystem is extensive, including, for example, enabling players as well as toolset and product developers. This calls for thorough research and due diligence.
In conjunction with ecosystem collaboration, players should establish relevant in-house capabilities. What these are and the depth of skills required clearly depend on the size of any investment, ranging from “intelligent buyer” at one end of the scale through to requiring full, in-house expertise at the other.
Build & maintain ecosystem presence
As always with developing technologies, the timing of growth rates and innovation breakthroughs are difficult to predict. Involvement and presence in the ecosystem are therefore key to responding rapidly if and when an application starts to see exponential growth. SynBio has shown recent accelerations that suggest it could very well achieve this exponential growth in the coming years.
Involvement in the ecosystem is also important to engage constructively in discourse around key ethical and regulatory issues.
Generally, application end users will have invaluable expertise and knowledge to offer in respect to markets, customers, and go-to-market strategies. This expertise is especially useful for technology and product developers, many of which will be start-ups and may come from an academic or technical perspective.
Conclusion — Companies need to be ready for growth
Overall, the SynBio market faces significant challenges to success. To better understand these, our 2023 global expert survey ranked known SynBio challenges in terms of their importance/criticality and the level of uncertainty around whether they would be overcome in five to 10 years (see Figure 28).
Survey responses show a large number of challenges that combine high criticality and high uncertainty, including:
-
Overcoming key skills and competency gaps (including genetic-editing capabilities)
-
Achieving standardized toolsets and systematizing the knowledge base
-
Continued availability of funding and investment
-
Negative public perception and overcoming ethical and biosecurity concerns
-
Meeting the challenges of scaling up for industrial-level manufacturing
-
The availability of big (biological) data
- The challenge of better DNA synthesis tools (#7) was also considered critical but was considered more likely to be overcome within the five- to 10-year time frame. Other important challenges with high uncertainty included:
-
IP/technology ownership challenges
-
Overcoming fundamental technology and scientific challenges
-
Regulation and policy barriers
-
Insufficient market demand (due to poor economics)
-
Lack of coordinated programs and open innovation
- Compute power and speed of simulations (#13) is also an important enabler but was judged more likely to be achieved within the time frame.
In conclusion, the challenges to the successful growth of the SynBio market are significant and wide ranging. There are many critical uncertainties and, in many SynBio applications, development has been ongoing for decades, often driven more by technology push than market pull.
However, the potential SynBio offers is equally strong, and companies should understand that:
-
SynBio is an extremely diverse field. SynBio is fairly unique in the breadth of potential applications — from healthcare to food, to manufacturing, to industrial processing, and even to the digital industries. Its technologies range from the embryonic through to the fully commercialized. In considering its prospects, it is therefore important to take a sufficiently granular approach, based on the field.
-
Many SynBio applications are poised for growth. Forecast growth rates for SynBio are >20% CAGR to 2030 overall, especially in healthcare and life sciences and food and agriculture. Drivers for growth are strong, both on the supply side (increasing investment, lower technology costs, growing ecosystems) and the demand side (e.g., sustainability, healthcare needs, manufacturing efficiency).
-
SynBio technologies could have a massive transformational impact in a 20-year time frame. Technologies such as predictive genetic engineering, DNA synthesis for complex organisms, DNA-based storage, and AI-powered applications could transform industries, our ability to engineer living systems, and how we cope with climate change.
All this means that companies should ensure they have a suitable SynBio strategy in place. Given its diversity, companies must consider carefully which specific fields are relevant for their business and conduct realistic market-based assessments. At the same time, due to the strong drivers, accelerating technology developments, and potentially huge impacts, companies should be prepared for exponential growth that could result from breakthroughs as we move toward a SynBio world.
Appendix 1
Example SynBio tool & technology players
Appendix 2
Example SynBio application players
Notes
[1] Gallagher, James. “Scientists Grow Whole Model of Human Embryo, Without Sperm or Egg.” BBC News, 6 September 2023.
[2] Survey comprised responses from 38 academic and industry leaders from around the world.
[3] Hybrid: systems that combine organic and inorganic materials.
[4] Wang, Bo, et al. “Synthesis and Enhanced Oil Recovery Potential of the Bio-Nano-Oil Displacement System.” ACS Omega, Vol. 8, No. 19, May 2023.
DOWNLOAD THE FULL REPORT