24 min read • Automotive
Demystifying the future of hydrogen mobility in India
Exploring the potential of hydrogen to fuel India’s growth
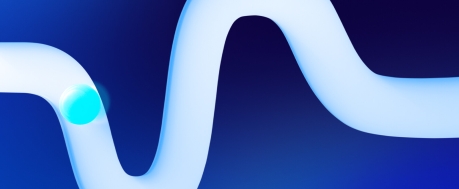
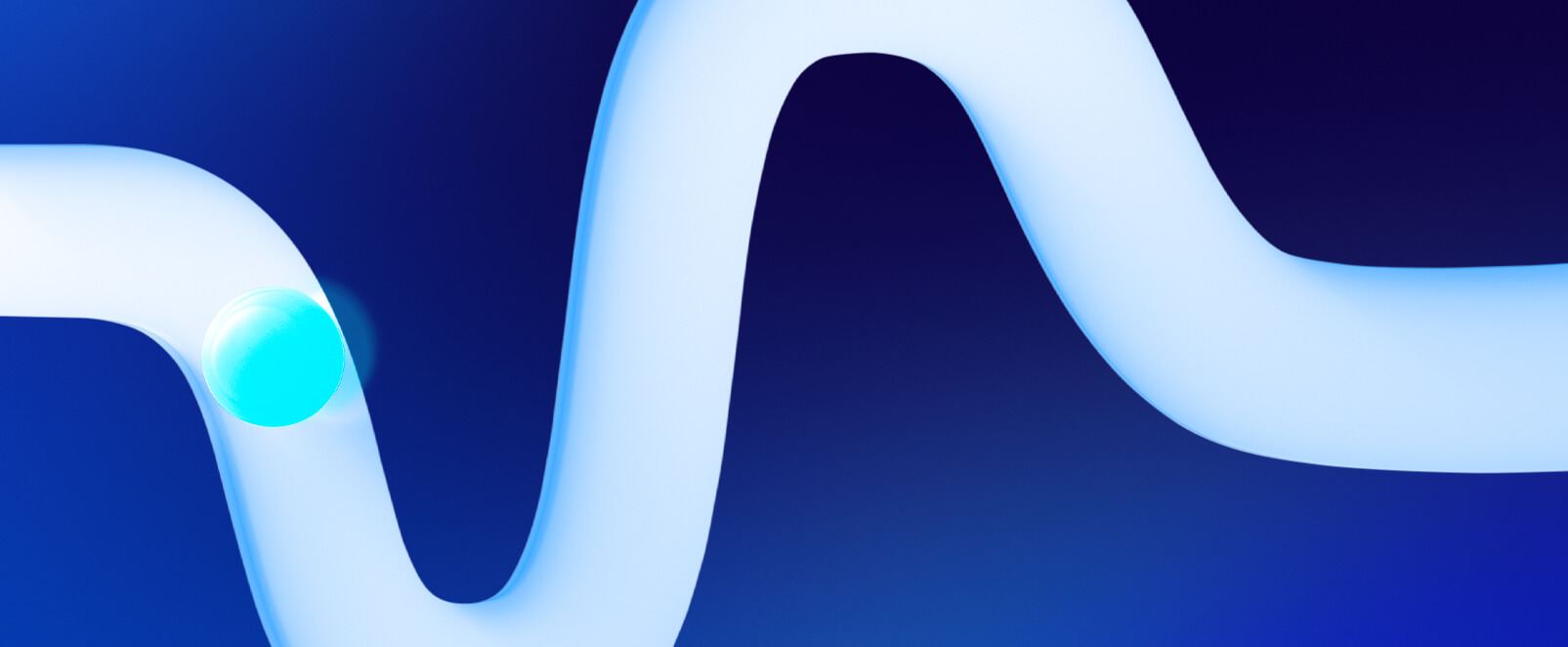
Executive Summary
E-mobility has occupied a prominent position in discussions centered on the future of transportation. During the last decade, battery-powered electric vehicles (BEVs) have become increasingly popular as sustainable mobility is advocated for worldwide. However, BEVs are plagued by their lack of renewable power and the strain they put on limited natural resources. These problems create a gap, which sustainable fuel can fill.
Clean fuel and hydrogen, an abundant natural resource, have substantial potential in this space, which could be realized through fuel cell electric vehicles (FCEVs). FCEVs use a fuel cell to generate electricity from compressed gaseous or liquid hydrogen and do not produce harmful tailpipe emissions. A variety of use cases featuring FCEVs are emerging globally, and the implications of this technology and the growth opportunities it presents to India need to be explored.
During the 21st century, various stakeholders have invested concrete efforts to get hydrogen-fueled vehicles on the road. Hyundai, Honda, and Toyota each launched FCEVs, and the technology gained some initial traction; however, its rise has been dwarfed by the rise of battery electric vehicles. Our analysis of both technologies suggests that FECVs are suited to heavy-duty vehicles while BEVs are more suited to passenger vehicles. Therefore, instead of competing, the two technologies can complement each other to drive the growth of e-mobility. In this Report, we evaluate the unique value proposition of FCEVs and assess their relevance in India’s rapidly growing e-mobility space.
Governments worldwide have different outlooks on hydrogen’s prospects for mobility. Because the technology is still in its initial stage, government support and policies to protect and prioritize advancement will have a crucial impact on its adoption. In this Report, we also analyze initiatives taken by different countries and their governments, driven by different visions on using hydrogen for mobility. We take a deep dive into two countries where government support has enabled hydrogen mobility to advance quickly.
Rapid innovation in the BEV industry (including improved battery capacities, charging speeds, and technology costs) increased awareness of the need for sustainable mobility, and government support has spurred consumer interest and improved the trustworthiness of the technology. In this optimistic scenario for BEVs, it is crucial to understand what might drive FCEV growth and how the two technologies can coexist. We highlight complementary value propositions, identify favorable use cases for both technologies, and delve into different hydrogen categories based on their source and their resulting ecological footprint. We provide a glimpse into alternative use cases for hydrogen in mobility beyond FCEVs.
Lastly, let’s steal a peek into the future of hydrogen mobility in India. India’s mobility space will likely see a surge in new technologies that may compete with FCEVs; however, each newcomer will represent added value for the journey toward sustainable mobility. Keeping a balanced outlook for policy making and industrial growth will provide a level playing field for all technologies.
1
THE ADVENT OF HYDROGEN IN E-MOBILITY
THE HISTORY & THE JOURNEY TO FCEVs
Advancements toward sustainability goals and the resulting demand for cleaner fuels have sparked consumer’ interest in e-mobility, while gaining support from industry players and governments worldwide. Global electric car sales reached a record high in 2021 despite supply chain bottlenecks and the ongoing COVID-19 pandemic. Compared to 2020, the International Energy Agency (IEA) reports sales nearly doubled to 6.6 million (a sales share of almost 9%), bringing the worldwide total of electric cars on the road to 16.5 million.[1]
As the world switches to electric vehicles, research continues to seek methods to power electric cars with the most abundant resource in the universe: hydrogen. On earth, hydrogen is available primarily in molecular form and needs to be harnessed through industrial processes, the sustainability of which is indicated through a kaleidoscope of colors:
-
Green hydrogen is produced through electrolysis, using clean energy from renewable sources.
-
Blue hydrogen is generated by subjecting natural gas to steam reforming, while the resulting CO2 is captured and stored using carbon-capture and storage technology.
-
Gray hydrogen production is similar to blue hydrogen, but it eliminates the carbon-capture and storage step, making it less environmentally friendly than blue.
-
Black or brown hydrogen is processed from coal and constitutes an ecologically unfriendly way of generating hydrogen.
Natural gas accounts for 48% of industrial hydrogen production, while 18% is sourced from coal.[2] As of 2017, India ranks 22nd globally for industrial hydrogen production, holding 43 trillion cubic feet of proven gas reserves and making hydrogen a comparatively accessible fuel.[3] While hydrogen has multiple use cases for e-mobility, we will focus on FCEVs, which are based on the technology combining hydrogen and oxygen in a fuel cell to produce electricity that powers the electric motor to drive the car. FCEVs have emerged as an alternative to battery-powered electric vehicles due to their fast refueling capabilities, extended range potential (given hydrogen’s high gravimetric energy density), and lack of dependence on concentrated rare raw materials.
In 2013, Hyundai Motor Group introduced to the world the first commercially produced hydrogen fuel cell automobile, the ix35 FCEV. Honda had a vehicle named Clarity, and Toyota entered the market in 2014 by launching Mirai. Hyundai plans to offer hydrogen fuel cell versions for all its commercial vehicles by 2028. Figure 1 shows a timeline of other significant milestones in the rise of hydrogen’s contributions to mobility.
Although they are in their initial stage, FCEVs are poised for growth. The volume breakthrough is not expected to happen until the second half of this decade, with Asia leading the charge (see Figure 2). Significant government and private investments will be required for the breakthrough of FCEVs.
2
WHERE THE WORLD STANDS ON HYDROGEN MOBILITY
GLOBAL USE CASES & IMPLEMENTATION STRATEGIES FOR HYDROGEN MOBILITY
The global hydrogen fuel cell vehicle market size was estimated at almost US $1 billion in 2021 and is expected to hit around US $43.2 billion by 2031, registering a significant CAGR of 45.5% from 2022 to 2031.[4] Asian Pacific countries are the largest market for fuel cell technology, with Japan, China, and South Korea paving the way forward; Europe and North America lag behind.[5]
China, exploring the potential of FCEVs[6] launched its first mass-produced passenger FCEV in 2022, the Changan Shenlan SL03, after deploying significant numbers of heavy-duty FCEVs. The US market has witnessed the growing adoption of FCEVs, with 3,341 units sold during 2021.[7]
Chicago, Vancouver, London, and Beijing are among the major cities experimenting with hydrogen-powered buses. Hydrogen fuel cell trains have been launched in Germany and are expected to appear across Europe and the US over the next five years.
Some developing nations, while not looking at hydrogen for mobility applications, are currently investing heavily in hydrogen energy. Net energy importers like Chile, South America, and African countries such as Morocco and Namibia are emerging as exporters of emissions-free green hydrogen, generated using renewable energy. Meanwhile, fossil fuel exporters like Australia, Oman, Saudi Arabia, and the United Arab Emirates are seeking clean hydrogen to help diversify their economies.
Thirteen million FCEVs are estimated to be sold globally by 2030, of which 5.1 million will be in Asia alone.[8] Policy support for hydrogen deployment worldwide is tied to current growth across vehicle types.
JAPAN STEPS TOWARD A HYDROGEN ECONOMY
Japan is striving to decarbonize its economy by utilizing hydrogen energy in transportation, manufacturing, power generation, and other areas. It published the Basic Hydrogen Strategy in 2017, making Japan the first country to establish a national hydrogen framework. Growth in the passenger FCEV market over recent years has reflected the Japanese government’s efforts to propagate hydrogen technology (see Figure 3). Toyota, Japan’s largest automaker, placed its trust in hydrogen as an alternative energy source for transportation and led the way in fuel cell vehicle market expansion with its 2014 launch of the Mirai passenger car.
Japan has also set sales targets for FCEVs, aiming for 200,000 units by 2025 and 800,000 units by 2030.[9] The government has developed initiatives to make FCEVs affordable and competitive. Technological developments, public-private coordination, and regulatory frameworks support this planning. The following examples of these initiatives depict Japan’s faith in FCEV technology and green hydrogen’s importance to e-mobility:
-
Supporting energy R&D. The New Energy and Industrial Technology Development Organization (NEDO) oversees the Zero Emissions Creators 500 program. It seeks to assist 500 young energy and environmental researchers at universities over a five-year period by connecting them with corporate research institutes and other organizations and entities.
-
Establishing hydrogen supply chains. Japan has initiated pilot projects to import hydrogen by developing international hydrogen supply chains. Projects with Saudi Arabia, Australia, and Brunei are underway, which focus on extracting and importing different forms of hydrogen.
-
Developing infrastructure for FCEVs. Japan intends to set up 320 hydrogen refueling stations. To control construction and operating costs, cost targets have been established with government aid in the form of subsidies and required changes to regulations.
Japan aims to grow its hydrogen market from 2 million tons per year to 3 million tons per year by 2030 and 20 million tons per year by 2050. The government also hopes to reduce current hydrogen costs by 2030 to one-third of what they are now. Heavy R&D, demonstration, and deployment investments are being made to fulfill these goals.[10]
GERMANY INVESTS IN ITS HYDROGEN SECTOR
To decarbonize its industry and speed up its energy transition, Germany plans to invest roughly €3.6 billion EUR (approximately US $3.75 billion) to boost the green hydrogen sector.[11] The country will establish a governance structure, develop international partners for hydrogen, and focus primarily on green hydrogen. The State Secretaries’ Committee on Hydrogen appointed 25 members to the German National Hydrogen Council representing business, science, and civil society interests. The council provides suggestions and recommendations for action to help the State Secretaries’ Committee implement and improve its hydrogen strategy. The Committee also plans to import green hydrogen by building infrastructure in alliance nations. Germany is expected to invest in a 100 MW green hydrogen facility in Morocco.[12]
Hydrogen usage in Germany is expected to rise across categories, as it transitions to clean energy and drives the growth of mobility use cases for hydrogen. The country announced a blueprint for the world’s largest hydrogen grid, covering about 5,900 km, and the first phase of 1,200 km will be ready by 2030.[13] The country took an ingenious leap by establishing infrastructure for hydrogen transmission, by developing a strategy of repurposing existing gas pipelines for the hydrogen grid. During the first phase, only 100 km of the grid will be newly built; the rest of the grid will consist of repurposed gas pipelines. Free transmission through the gas pipeline network has been provided to promote green hydrogen. This will establish a strong hydrogen mobility infrastructure base to support EV growth. Germany’s H2 Mobility fueling station network will get an extra €110 million EUR (US $121 million) over the next five years to support the mobility use case by rolling out more infrastructure for hydrogen-powered fuel cell vehicles.[14]
LESSONS FOR THE INDIAN MARKET
Japan and Germany showcase the key role that hydrogen can play in the energy transition. Hydrogen shows the most significant potential for long-range applications or applications with significant auxiliary loads (e.g., refrigeration) in mobility and transport. Large passenger vehicles, buses, commercial vehicles, off-highway vehicles, rail, marine, and aircraft provide promising mobility and transport use cases. Hydrogen also provides energy-storage capabilities; green hydrogen can, in fact, serve as a storage medium for intermittent and often remotely generated renewable energy. Both countries understand that multiple “chicken and egg” problems must be resolved on both the supply and demand sides to activate the market. Collaboration between the government, energy companies, and OEMs is required to establish the hydrogen generation, distribution, and refueling infrastructure. Joint efforts from the policymaking and industry sides are critical for the adoption of hydrogen mobility.
3
INDIA’S FORAY INTO HYDROGEN MOBILITY
SUSTAINABILITY TARGETS SHIFTED INDIA’S FOCUS TO GREEN HYDROGEN
Hydrogen’s potential in the mobility space could fuel India’s journey toward “Atmanirbhar Bharat” — a self-reliant India. As EV adoption increases, India’s dependence on imported lithium and other rare elements also increases. India has set a goal to make its e-mobility industry energy-independent by 2047, and hydrogen can be vital to supporting the country’s aspirations. As part of the effort to move toward this goal, the Union Cabinet of India approved the eagerly anticipated National Green Hydrogen Mission on 4 January 2022.
Mission outcomes
The mission’s outcomes include the development of a green hydrogen production capacity of at least 5 million metric tons (MMT) per annum with an associated renewable energy capacity addition of about 125 gigawatts (GW) in the country. In addition, the mission aims to make India a leading global producer and supplier of green hydrogen. The Union Cabinet made an initial investment in the National Green Hydrogen Mission of Rs 19,744 crore (over US $2 billion).[15] This mission thrusts India onto the path to sustainability, as nearly 50 MMT per annum of greenhouse gas (GHG) emissions are expected to be averted by 2030.[16] The Ministry of New and Renewable Energy has moved a cabinet note outlining the mission’s requirements for R&D and green hydrogen consumption obligations. The Indian government’s vision is reflected by its support for the hydrogen-powered FCEV Toyota Mirai.
Investments by the Indian government
The government has designated Rs 17,490 crore (88.6%) of the mission’s total budget for incentives to generate green hydrogen and manufacture electrolyzers, which it has dubbed the Strategic Interventions for Green Hydrogen Transition (SIGHT) Programme. The National Green Hydrogen Mission is expected to attract a total investment of Rs 800,000 crore by 2030 and create around 600,000 jobs.[17]
Impact on industry
Heavy-duty trucks are expected to be significant in the hydrogen mobility space, although they are not forecasted to be on the road until the latter part of this decade. By 2030, it is estimated that 12,000 FCEV heavy-duty trucks will be running on Indian roads.[18] In our earlier Viewpoint, “Hydrogen: Trucking’s Alternative Fuel of the Future,” we discussed how heavy-duty vehicles generate approximately one-third of GHG emissions, even though these comprise only a small number of vehicles on the road. This statistic highlights the need to replace heavy-duty vehicles with FCEVs.
The government’s initiatives have helped build trust among the private sector. Tata Motors has signed an agreement with Cummins, Inc., a global provider of power solutions and hydrogen technologies, to collaborate on designing and developing low- and zero-emission propulsion technology solutions for commercial vehicles in India. This collaboration would include hydrogen-powered internal combustion engines (ICEs), fuel cells, and battery electric vehicle systems. Agreements between companies, like the one between Tata Motors and Cummins paint a positive picture and promote the National Green Hydrogen Mission as a great launchpad for FCEVs in India.
4
COMPARING FCEVs & BEVs
HOW DO FCEVs HOLD UP AGAINST THE COMPETITION?
FCEVs have a strong and popular competitor in BEVs, which have a tight grasp on the market. To evaluate the viability of FCEVs, we have compared them to BEVs on seven vital parameters: (1) driving range, (2) refueling time, (3) infrastructure, (4) hydrogen permeation, (5) energy efficiency, (6) environmental impact, and (7) total cost of ownership (TCO). The following analysis aims to assess the strengths and the best use cases for each technology.
Driving range
Since their origin, consumers have associated electric vehicles with “range anxiety,” which is the fear that the vehicle’s battery will not have enough range to travel the desired distance. BEVs have evolved significantly to improve their ranges but fall short of the range potential of FCEVs (see Figure 4).
The average range of an FCEV is 483 km, compared to the 322 km range offered by a BEV. The Toyota Mirai FCEV provides a range of 650 km, while only a few high-end BEVs can deliver around 565 km.
When examining the driving range, the weight-range trade-off is a crucial aspect to include. FCEVs fare much better than BEVs on this parameter. When more range is added to an FCEV, the weight increase is marginal. It is significantly less than a range increase in a BEV; here, the battery weight goes up far more steeply as the range increases. Hydrogen has a high gravimetric energy storage density, meaning the amount of energy stored per unit mass is 120 megajoules per kilogram (MJ/kg). The weight advantage of energy storage of an FCEV, compared to a BEV, translates to significant payload improvements for commercial vehicles.
Refueling time
FCEVs pull far ahead of BEVs on this metric, requiring just a few minutes to fully refuel. In contrast, BEVs typically need 30 minutes to attain an 80% charge, which requires fast charging capability on both the vehicle and infrastructure sides. Otherwise, refueling could take multiple hours. However, fast charging typically comes at a higher cost per kWh and has a negative impact on battery life.
Innovations in BEVs have the potential to reduce charging time drastically, providing 80% charging in around 15 minutes. Tesla’s super-fast chargers promise a 300 km range in 15 minutes of charge time. However, these are only available for high-end vehicles and haven’t yet been tested in the Indian market. Significant investment in India’s grid infrastructure would be required to support a fast-charging infrastructure deployment. Therefore, the economic and commercial viability of these innovations in BEVs is unknown.
Infrastructure
BEV charging infrastructure is far more developed than the refueling infrastructure for FCEVs. But both technologies face challenges to establishing the infrastructure they need to keep up with demand. Figure 5 shows the availability and type of publicly accessible EV chargers, by country, while Figure 6 shows available hydrogen fueling stations by country.
Let’s look at the key challenges FCEVs and BEVs must overcome as they grow their infrastructure:
-
High initial setup costs. Establishing the infrastructure for FCEVs requires a more considerable initial investment than BEVs. The cost of constructing a hydrogen refueling station is roughly US $2 million (spent by both Columbia and the US), which is substantially higher than an electric charging station (US $50,000) or a gas or petrol station.[19] The nascent stage of FCEV adoption has caused concern for companies that are uncertain about their return on investment.
-
Safety concerns. Hydrogen is highly combustible, hard to store and transport, and necessitates advanced technology to safely establish supply chain systems. Truck transportation of hydrogen, storage at hydrogen stations, and the corresponding dispensing units need to follow high safety standards, which might be a financial burden on initial investments and regular operating costs. In comparison, BEV charging stations are known to be relatively safe, given the higher level of maturity of charging technologies and software.
-
Real estate requirements. Hydrogen stations are difficult to build and operate due to safety concerns and the need for specialized storage tanks. They must also adhere to strict safety standards, while BEV charging stations feature multiple chargers and can be installed near offices and businesses. Ideally, BEV charging stations should be located near a grid that can simultaneously withstand the expected load from multiple chargers connections.
Hydrogen permeation
Hydrogen permeation is another consideration when dealing with FCEVs. This refers to the process of hydrogen gas passing through a solid material due to the difference in the partial pressures of hydrogen on either side of the material. It is deliberately allowed in some hydrogen fuel cells to prevent the buildup of hydrogen pressure inside the cell, which could lead to a rupture. The amount of hydrogen allowed to permeate is carefully controlled to ensure that it does not cause a significant loss of fuel. Monitoring the amount helps keep the concentration of hydrogen in the air below the flammable limit. BEVs face no comparable leakage issue.
Energy efficiency
Energy efficiency is crucial to a vehicle’s TCO, usage flexibility, and sustainability. The well-to-wheel energy efficiency of hydrogen FCEVs is significantly lower than BEVs. The FCEV has a well-to-wheel energy efficiency of 25%-35%, while the BEV has a well-to-wheel efficiency of 70%-80%. Figure 7 shows how the two compare on this measure.
With regard to storable energy, FCEVs benefit from an inherently high gravimetric energy density of 33.6 kilowatt-hours per kilogram (kWh/kg) of hydrogen. This is much lower than the energy density of electric batteries, which is approximately 250 kWh/kg, as currently used by Tesla. However, hydrogen’s low volumetric energy density in compressed gaseous and liquid form constrains the realistically storable content in a vehicle, and the weight of the hydrogen storage tanks offset some of the gravimetric benefits.
Environmental impact
The ecological efficiency of FCEVs and BEVs is determined by their energy source and production. The reputation of BEV manufacturing is marred by environmental damage caused by mining for raw materials concentrated in certain parts of the world, making battery production the most questionable part of EV production. In our Viewpoint, “Toward a Sustainable Value Chain for Electric Vehicles in India,” we delve deeper into the social and environmental risks associated with mining battery raw materials.
Today, almost all hydrogen is derived from carbon-based materials with significant CO2 emissions. Like the earlier classification, which assigns hydrogen a color based on its level of sustainability, this approach divides hydrogen into five categories based on production process. The criteria for each color could change over time and may even differ among different countries, as there is no universal naming convention.
As discussed in the “Energy efficiency” section above, well-to-wheel efficiency is substantially higher for BEVs. FCEVs use ecologically inefficient methods of hydrogen production, allowing BEVs to take the lead in environmental friendliness.
However, FCEVs are catching up with innovative solutions. According to Toyota, its FCEV Mirai cleans the air and is a negative-emission vehicle, capturing even fine particulate matter (PM) 2.5 particles. Air goes through the car’s numerous membranes, ultimately flushing cleaner air back into the atmosphere.
Green hydrogen production will make hydrogen more environmentally friendly, even though the efficiency of the process might still be lacking. FCEVs show great potential to create a positive impact on the environment.
TCO analysis
We arrived at the TCO by considering the purchase price, the running cost of fuel, and the subsequent cost of operations and maintenance (O&M) undertaken by a typical customer (see Table 1). The purchase price is the average one-time cost to buy the car. O&M costs include maintenance, parts replacement, expected depreciation, insurance, and other expenses. We have used the average prices of standard passenger cars for this comparison (see Figure 8).
As economies of scale and government subsidies kick in, we expect the TCO for both BEVs and FCEVs to decrease. Therefore, for FCEV passenger cars, we included the future forecasted costs. We also benchmarked both BEVs and FCEVs against ICE vehicles, assuming ICEs have reached full economies of scale and have a fully developed supporting infrastructure.
The following assumptions are crucial to our analysis:
-
Our TCO calculations were based on a six-to-eight-year vehicle lifespan and an assumed total distance traveled by the car of 150,000 km.
-
We used current energy prices and assumed they would remain constant.
-
Our estimate of the cost of hydrogen fuel was based on conservative industry forecasts provided by experts.
-
Currently, the Toyota Mirai is the only hydrogen FCEV available in India. However, we anticipate more models becoming available in the coming years by different manufacturers; this will drive down prices due to competition, government support, and economies of scale. Therefore, for comparison purposes, we estimated the cost of a more affordable version of FCEVs.
Despite the higher initial cost of both BEVs and FCEVs when compared to ICEs, the lower running expenses of both types of EVs will allow for the recovery of the initial cost over the vehicle’s lifespan. The high initial cost for FCEVs will also decrease, making them more attractive in terms of TCO.
FCEVs’ costs will decrease over the next decade, benefiting from economies of scale and technological innovations. Hydrogen fuel costs are expected to decline; creating the required infrastructure will support the growth of FCEVs, and OEMs will compete over the consumer base. When compared to BEVs and ICEs, these factors will support the growth of FCEVs and offer unique value propositions and cost-effectiveness.
5
ALTERNATIVE MOBILITY USES FOR HYDROGEN
HYDROGEN CAN ENABLE A SMOOTH TRANSITION TO EVs
Hydrogen has numerous on-the-ground use cases; many are already being implemented globally. Countries like Germany are primarily focused on FCEVs; however, India is also pursuing alternatives that provide opportunities for rapid uptake of hydrogen and partial use of legacy technology. Vehicle manufacturers could pick these up quickly to extend existing technology for longer, using cleaner fuel alternatives. End users will rely more on tried-and-tested technology to smooth the transition, and alternatives will be more suitable for heavier loads and other requirements. These steps will require setting up a hydrogen ecosystem that could also be used for FCEVs. There are prominent examples of these alternatives, including:
-
Hythane (H-CNG). H-CNG is a hydrogen-enriched compressed natural gas (CNG). It is cleaner, more cost-efficient, and increases fuel efficiency. With 70% more reduction in carbon monoxide emissions compared to CNG, H-CNG could be an essential solution to fulfill upcoming emission requirements. The Indian Oil Corporation Limited (IOCL), in collaboration with the Delhi Transport Department, set up a compact reformer-based plant capable of producing 4 tons of H-CNG a day.
-
Methanol (H2 derived) blending of gasoline. Methanol can be processed to make dimethyl ether (DME), which would be a cleaner substitute for diesel and would only require slight changes to existing diesel engines. According to a NITI Aayog report, blending 15% methanol with gasoline can reduce India’s import of crude oil by about 15%. This blend would also help improve air quality by reducing GHG emissions by 20%. IOCL rolled out M15 petro, a 15% blend of methanol with petrol, as part of a pilot project in Assam’s Tinsukia district.
-
Ammonia (H2 derived) for ships. Ammonia is another cleaner alternative that can be used by fuel cells and ICEs. It can be made using renewable resources and has easy storage options. Ammonia is a zero-carbon option with lower costs than its counterparts. Its high energy density and scalability have made it one of the optimum choices for marine fuel. A German multinational company, MAN Energy Solutions, has developed a timeline to deliver commercially viable ammonia-burning engines by 2024. It aims for economically feasible and scalable solutions that could be rapidly deployed.
-
H2 ICE. This entails substituting petrol, diesel, or CNG with hydrogen in a vehicle with an ICE. H2 ICE will require familiar parts from vehicle manufacturers and provide end users with reliable and trusted technology. This change will translate to zero emission of carbon-based pollutants. However, since hydrogen is burned at high temperatures with ambient air, nitrogen oxide emissions will generate pollution. Still, H2 ICE would have zero-carbon tailpipe emissions and be able to share hydrogen production and distribution ecosystem infrastructure with both ICEs and FCEVs. In India, Tata Motors aims to use H2 ICE technology for its buses and trucks to leverage ICE’s intellectual property and infrastructure.
6
WHAT THE FUTURE HOLDS FOR INDIA
GROWTH IN HYDROGEN MOBILITY CAN ACHIEVE ITS SUSTAINABILITY GOALS
Dependence on imports is a critical constraint for existing BEV models. FCEVs provide the opportunity to decrease this reliance, which supports the Indian government’s Atmanirbhar Initiative. Steps taken in this direction will work toward fulfilling an essential element of India Vision 2030, which is pivotal to the transportation ecosystem: a pollution-free India.
The entrance of FCEVs in a fast-evolving mobility space may create uncertainties in OEM strategies and in customers’ minds. Therefore, the government should play a vital role in facilitating a smooth transition within the car sector to allow these various technologies to coexist. One possible strategy is to introduce FCEVs for buses, trucks, and other heavy-duty vehicles, while BEVs remain exclusive to the passenger car category, where they already dominate the EV race and would continue to thrive. This approach could be viable, as establishing infrastructure for FCEVs would take less time and investment because fewer stations and establishments would be required than for passenger vehicles. Hydrogen’s high gravimetric energy density is more suitable for long-distance, heavyweight, and auxiliary load mobility and transport, as discussed earlier in the report. Our TCO analysis also supports this hypothesis. Based on the above analysis and our TCO calculations, we anticipate a production timeline of different vehicle types across use cases as reflected in Figure 9.
As FCEVs carve out their mobility and transport space niche, the government needs an effective strategy to build a sustainable ecosystem around hydrogen-powered vehicles and ensure a smooth transition for the auto industry. From a policy-making perspective, the following actions can help drive the growth of FCEVs in the Indian market:
-
The government can provide subsidiaries and incentives for building the necessary infrastructure (from converting green energy to hydrogen, to building hydrogen fuel stations) to support the growth of hydrogen vehicles.
-
Private players, companies, and research institutions should be encouraged to invest in R&D for hydrogen in mobility and identify ways to improve its cost-effectiveness.
-
The government can encourage near-term market development by identifying industrial clusters and enacting viability gap funding and mandates.
FCEVs can save OEMs from the sudden downfall of existing technology and a demand crisis, especially for commercial vehicles where battery technology is still not advanced and sustainable targets are not getting any closer. Therefore, the government’s proactive support for the growth of technology could prove crucial in defining the future of India’s mobility space.
Notes
[1] “Global Electric Car Sales Have Continued Their Strong Growth in 2022 After Breaking Records Last Year.” Press release, International Energy Agency (IEA), 23 May 2022.
[2] Baharudin, Luqmanulhakim, and Matthew Watson. “Hydrogen Applications and Research Activities in Its Production Routes Through Catalytic Hydrocarbon Conversion.” Reviews in Chemical Engineering, Vol. 34, No. 1, March 2017.
[3] “2023 Global Threat Report.” CrowdStrike, 2023.
[4] T., Ashish, and Sonia M. “Hydrogen Fuel Cell Vehicle Market by Vehicle Type (Sedan, SUV, Others), by Technology (Proton Exchange Membrane Fuel Cell, Phosphoric Acid Fuel Cell), by Range (0-250 Miles, 251-500 Miles, Above 500 Miles): Global Opportunity Analysis and Industry Forecast, 2021–2031.” Allied Market Research, December 2022.
[5] McDonald, Jeffrey. “Fuel Cell EVs Set to Top 13 Million by 2030 as Hydrogen Scales Up: Hydrogen Council.” S&P Global Commodity Insights, 24 January 2020.
[6] Creamer, Martin. “China Becoming Fuel Cell Electric Vehicle Leader, World Platinum Research Indicates.” Mining Weekly, 20 October 2022.
[7] “Analysis of Fuel Cell Vehicle Market in North America — Growth, Trends, and Forecasts (2023-2028).” Mordor Intelligence, accessed May 2023.
[8] McDonald, Jeffrey. “Fuel Cell EVs Set to Top 13 Million by 2030 as Hydrogen Scales Up: Hydrogen Council.” S&P Global Commodity Insights, 24 January 2020.
[9] Nakano, Jane. “Japan’s Hydrogen Industrial Strategy.” Center for Strategic & International Studies, 21 October 2021.
[10] “Japan Aims to Boost Hydrogen Supply to 12 Million T by 2040.” Reuters, 4 April 2023.
[11] “Germany Plans to Invest EUR 3.6 Billion in Green Hydrogen.” Power Info Today, accessed May 2023.
[12] Menon, Anoop. “Morocco’s First Green Hydrogen Project to Start Production in 2025.” ZAWYA, 24 May 2021.
[13] Martin, Nik. “Germany Invests €9 Billion in Hydrogen.” Deutsche Welle, 10 June 2020.
[14] “H2 Mobility Gets $121 Million from Investors to Build Hydrogen Network.” Reuters, 29 March 2022.
[15] Dutta, Sanjay. “Rs 19,744 Crore Incentive Makes India Global Green Hydrogen Hotspot.” Times of India, 5 January 2023.
[16] Ministry of New and Renewable Energy. “Cabinet Approves National Green Hydrogen Mission.” Press Information Bureau, Government of India, 4 January 2023.
[17] “Cabinet Approves National Green Hydrogen Mission.” Ministry of New and Renewable Energy, Government of India, 4 January 2023.
[18] “India’s Energy Transition Towards a Green Hydrogen Economy.” FTI Consulting, 9 June 2021.
[19] Melaina, M., and M. Penev. “Hydrogen Station Cost Estimates: Comparing Hydrogen Station Cost Calculator Results with Other Recent Estimates.” National Renewable Energy Laboratory, September 2013.
DOWNLOAD THE FULL REPORT
24 min read • Automotive
Demystifying the future of hydrogen mobility in India
Exploring the potential of hydrogen to fuel India’s growth
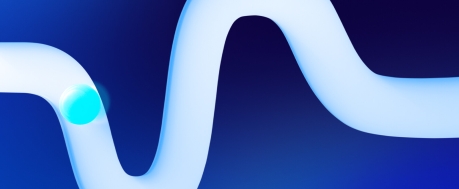
DATE
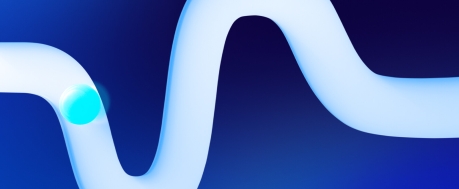
Executive Summary
E-mobility has occupied a prominent position in discussions centered on the future of transportation. During the last decade, battery-powered electric vehicles (BEVs) have become increasingly popular as sustainable mobility is advocated for worldwide. However, BEVs are plagued by their lack of renewable power and the strain they put on limited natural resources. These problems create a gap, which sustainable fuel can fill.
Clean fuel and hydrogen, an abundant natural resource, have substantial potential in this space, which could be realized through fuel cell electric vehicles (FCEVs). FCEVs use a fuel cell to generate electricity from compressed gaseous or liquid hydrogen and do not produce harmful tailpipe emissions. A variety of use cases featuring FCEVs are emerging globally, and the implications of this technology and the growth opportunities it presents to India need to be explored.
During the 21st century, various stakeholders have invested concrete efforts to get hydrogen-fueled vehicles on the road. Hyundai, Honda, and Toyota each launched FCEVs, and the technology gained some initial traction; however, its rise has been dwarfed by the rise of battery electric vehicles. Our analysis of both technologies suggests that FECVs are suited to heavy-duty vehicles while BEVs are more suited to passenger vehicles. Therefore, instead of competing, the two technologies can complement each other to drive the growth of e-mobility. In this Report, we evaluate the unique value proposition of FCEVs and assess their relevance in India’s rapidly growing e-mobility space.
Governments worldwide have different outlooks on hydrogen’s prospects for mobility. Because the technology is still in its initial stage, government support and policies to protect and prioritize advancement will have a crucial impact on its adoption. In this Report, we also analyze initiatives taken by different countries and their governments, driven by different visions on using hydrogen for mobility. We take a deep dive into two countries where government support has enabled hydrogen mobility to advance quickly.
Rapid innovation in the BEV industry (including improved battery capacities, charging speeds, and technology costs) increased awareness of the need for sustainable mobility, and government support has spurred consumer interest and improved the trustworthiness of the technology. In this optimistic scenario for BEVs, it is crucial to understand what might drive FCEV growth and how the two technologies can coexist. We highlight complementary value propositions, identify favorable use cases for both technologies, and delve into different hydrogen categories based on their source and their resulting ecological footprint. We provide a glimpse into alternative use cases for hydrogen in mobility beyond FCEVs.
Lastly, let’s steal a peek into the future of hydrogen mobility in India. India’s mobility space will likely see a surge in new technologies that may compete with FCEVs; however, each newcomer will represent added value for the journey toward sustainable mobility. Keeping a balanced outlook for policy making and industrial growth will provide a level playing field for all technologies.
1
THE ADVENT OF HYDROGEN IN E-MOBILITY
THE HISTORY & THE JOURNEY TO FCEVs
Advancements toward sustainability goals and the resulting demand for cleaner fuels have sparked consumer’ interest in e-mobility, while gaining support from industry players and governments worldwide. Global electric car sales reached a record high in 2021 despite supply chain bottlenecks and the ongoing COVID-19 pandemic. Compared to 2020, the International Energy Agency (IEA) reports sales nearly doubled to 6.6 million (a sales share of almost 9%), bringing the worldwide total of electric cars on the road to 16.5 million.[1]
As the world switches to electric vehicles, research continues to seek methods to power electric cars with the most abundant resource in the universe: hydrogen. On earth, hydrogen is available primarily in molecular form and needs to be harnessed through industrial processes, the sustainability of which is indicated through a kaleidoscope of colors:
-
Green hydrogen is produced through electrolysis, using clean energy from renewable sources.
-
Blue hydrogen is generated by subjecting natural gas to steam reforming, while the resulting CO2 is captured and stored using carbon-capture and storage technology.
-
Gray hydrogen production is similar to blue hydrogen, but it eliminates the carbon-capture and storage step, making it less environmentally friendly than blue.
-
Black or brown hydrogen is processed from coal and constitutes an ecologically unfriendly way of generating hydrogen.
Natural gas accounts for 48% of industrial hydrogen production, while 18% is sourced from coal.[2] As of 2017, India ranks 22nd globally for industrial hydrogen production, holding 43 trillion cubic feet of proven gas reserves and making hydrogen a comparatively accessible fuel.[3] While hydrogen has multiple use cases for e-mobility, we will focus on FCEVs, which are based on the technology combining hydrogen and oxygen in a fuel cell to produce electricity that powers the electric motor to drive the car. FCEVs have emerged as an alternative to battery-powered electric vehicles due to their fast refueling capabilities, extended range potential (given hydrogen’s high gravimetric energy density), and lack of dependence on concentrated rare raw materials.
In 2013, Hyundai Motor Group introduced to the world the first commercially produced hydrogen fuel cell automobile, the ix35 FCEV. Honda had a vehicle named Clarity, and Toyota entered the market in 2014 by launching Mirai. Hyundai plans to offer hydrogen fuel cell versions for all its commercial vehicles by 2028. Figure 1 shows a timeline of other significant milestones in the rise of hydrogen’s contributions to mobility.
Although they are in their initial stage, FCEVs are poised for growth. The volume breakthrough is not expected to happen until the second half of this decade, with Asia leading the charge (see Figure 2). Significant government and private investments will be required for the breakthrough of FCEVs.
2
WHERE THE WORLD STANDS ON HYDROGEN MOBILITY
GLOBAL USE CASES & IMPLEMENTATION STRATEGIES FOR HYDROGEN MOBILITY
The global hydrogen fuel cell vehicle market size was estimated at almost US $1 billion in 2021 and is expected to hit around US $43.2 billion by 2031, registering a significant CAGR of 45.5% from 2022 to 2031.[4] Asian Pacific countries are the largest market for fuel cell technology, with Japan, China, and South Korea paving the way forward; Europe and North America lag behind.[5]
China, exploring the potential of FCEVs[6] launched its first mass-produced passenger FCEV in 2022, the Changan Shenlan SL03, after deploying significant numbers of heavy-duty FCEVs. The US market has witnessed the growing adoption of FCEVs, with 3,341 units sold during 2021.[7]
Chicago, Vancouver, London, and Beijing are among the major cities experimenting with hydrogen-powered buses. Hydrogen fuel cell trains have been launched in Germany and are expected to appear across Europe and the US over the next five years.
Some developing nations, while not looking at hydrogen for mobility applications, are currently investing heavily in hydrogen energy. Net energy importers like Chile, South America, and African countries such as Morocco and Namibia are emerging as exporters of emissions-free green hydrogen, generated using renewable energy. Meanwhile, fossil fuel exporters like Australia, Oman, Saudi Arabia, and the United Arab Emirates are seeking clean hydrogen to help diversify their economies.
Thirteen million FCEVs are estimated to be sold globally by 2030, of which 5.1 million will be in Asia alone.[8] Policy support for hydrogen deployment worldwide is tied to current growth across vehicle types.
JAPAN STEPS TOWARD A HYDROGEN ECONOMY
Japan is striving to decarbonize its economy by utilizing hydrogen energy in transportation, manufacturing, power generation, and other areas. It published the Basic Hydrogen Strategy in 2017, making Japan the first country to establish a national hydrogen framework. Growth in the passenger FCEV market over recent years has reflected the Japanese government’s efforts to propagate hydrogen technology (see Figure 3). Toyota, Japan’s largest automaker, placed its trust in hydrogen as an alternative energy source for transportation and led the way in fuel cell vehicle market expansion with its 2014 launch of the Mirai passenger car.
Japan has also set sales targets for FCEVs, aiming for 200,000 units by 2025 and 800,000 units by 2030.[9] The government has developed initiatives to make FCEVs affordable and competitive. Technological developments, public-private coordination, and regulatory frameworks support this planning. The following examples of these initiatives depict Japan’s faith in FCEV technology and green hydrogen’s importance to e-mobility:
-
Supporting energy R&D. The New Energy and Industrial Technology Development Organization (NEDO) oversees the Zero Emissions Creators 500 program. It seeks to assist 500 young energy and environmental researchers at universities over a five-year period by connecting them with corporate research institutes and other organizations and entities.
-
Establishing hydrogen supply chains. Japan has initiated pilot projects to import hydrogen by developing international hydrogen supply chains. Projects with Saudi Arabia, Australia, and Brunei are underway, which focus on extracting and importing different forms of hydrogen.
-
Developing infrastructure for FCEVs. Japan intends to set up 320 hydrogen refueling stations. To control construction and operating costs, cost targets have been established with government aid in the form of subsidies and required changes to regulations.
Japan aims to grow its hydrogen market from 2 million tons per year to 3 million tons per year by 2030 and 20 million tons per year by 2050. The government also hopes to reduce current hydrogen costs by 2030 to one-third of what they are now. Heavy R&D, demonstration, and deployment investments are being made to fulfill these goals.[10]
GERMANY INVESTS IN ITS HYDROGEN SECTOR
To decarbonize its industry and speed up its energy transition, Germany plans to invest roughly €3.6 billion EUR (approximately US $3.75 billion) to boost the green hydrogen sector.[11] The country will establish a governance structure, develop international partners for hydrogen, and focus primarily on green hydrogen. The State Secretaries’ Committee on Hydrogen appointed 25 members to the German National Hydrogen Council representing business, science, and civil society interests. The council provides suggestions and recommendations for action to help the State Secretaries’ Committee implement and improve its hydrogen strategy. The Committee also plans to import green hydrogen by building infrastructure in alliance nations. Germany is expected to invest in a 100 MW green hydrogen facility in Morocco.[12]
Hydrogen usage in Germany is expected to rise across categories, as it transitions to clean energy and drives the growth of mobility use cases for hydrogen. The country announced a blueprint for the world’s largest hydrogen grid, covering about 5,900 km, and the first phase of 1,200 km will be ready by 2030.[13] The country took an ingenious leap by establishing infrastructure for hydrogen transmission, by developing a strategy of repurposing existing gas pipelines for the hydrogen grid. During the first phase, only 100 km of the grid will be newly built; the rest of the grid will consist of repurposed gas pipelines. Free transmission through the gas pipeline network has been provided to promote green hydrogen. This will establish a strong hydrogen mobility infrastructure base to support EV growth. Germany’s H2 Mobility fueling station network will get an extra €110 million EUR (US $121 million) over the next five years to support the mobility use case by rolling out more infrastructure for hydrogen-powered fuel cell vehicles.[14]
LESSONS FOR THE INDIAN MARKET
Japan and Germany showcase the key role that hydrogen can play in the energy transition. Hydrogen shows the most significant potential for long-range applications or applications with significant auxiliary loads (e.g., refrigeration) in mobility and transport. Large passenger vehicles, buses, commercial vehicles, off-highway vehicles, rail, marine, and aircraft provide promising mobility and transport use cases. Hydrogen also provides energy-storage capabilities; green hydrogen can, in fact, serve as a storage medium for intermittent and often remotely generated renewable energy. Both countries understand that multiple “chicken and egg” problems must be resolved on both the supply and demand sides to activate the market. Collaboration between the government, energy companies, and OEMs is required to establish the hydrogen generation, distribution, and refueling infrastructure. Joint efforts from the policymaking and industry sides are critical for the adoption of hydrogen mobility.
3
INDIA’S FORAY INTO HYDROGEN MOBILITY
SUSTAINABILITY TARGETS SHIFTED INDIA’S FOCUS TO GREEN HYDROGEN
Hydrogen’s potential in the mobility space could fuel India’s journey toward “Atmanirbhar Bharat” — a self-reliant India. As EV adoption increases, India’s dependence on imported lithium and other rare elements also increases. India has set a goal to make its e-mobility industry energy-independent by 2047, and hydrogen can be vital to supporting the country’s aspirations. As part of the effort to move toward this goal, the Union Cabinet of India approved the eagerly anticipated National Green Hydrogen Mission on 4 January 2022.
Mission outcomes
The mission’s outcomes include the development of a green hydrogen production capacity of at least 5 million metric tons (MMT) per annum with an associated renewable energy capacity addition of about 125 gigawatts (GW) in the country. In addition, the mission aims to make India a leading global producer and supplier of green hydrogen. The Union Cabinet made an initial investment in the National Green Hydrogen Mission of Rs 19,744 crore (over US $2 billion).[15] This mission thrusts India onto the path to sustainability, as nearly 50 MMT per annum of greenhouse gas (GHG) emissions are expected to be averted by 2030.[16] The Ministry of New and Renewable Energy has moved a cabinet note outlining the mission’s requirements for R&D and green hydrogen consumption obligations. The Indian government’s vision is reflected by its support for the hydrogen-powered FCEV Toyota Mirai.
Investments by the Indian government
The government has designated Rs 17,490 crore (88.6%) of the mission’s total budget for incentives to generate green hydrogen and manufacture electrolyzers, which it has dubbed the Strategic Interventions for Green Hydrogen Transition (SIGHT) Programme. The National Green Hydrogen Mission is expected to attract a total investment of Rs 800,000 crore by 2030 and create around 600,000 jobs.[17]
Impact on industry
Heavy-duty trucks are expected to be significant in the hydrogen mobility space, although they are not forecasted to be on the road until the latter part of this decade. By 2030, it is estimated that 12,000 FCEV heavy-duty trucks will be running on Indian roads.[18] In our earlier Viewpoint, “Hydrogen: Trucking’s Alternative Fuel of the Future,” we discussed how heavy-duty vehicles generate approximately one-third of GHG emissions, even though these comprise only a small number of vehicles on the road. This statistic highlights the need to replace heavy-duty vehicles with FCEVs.
The government’s initiatives have helped build trust among the private sector. Tata Motors has signed an agreement with Cummins, Inc., a global provider of power solutions and hydrogen technologies, to collaborate on designing and developing low- and zero-emission propulsion technology solutions for commercial vehicles in India. This collaboration would include hydrogen-powered internal combustion engines (ICEs), fuel cells, and battery electric vehicle systems. Agreements between companies, like the one between Tata Motors and Cummins paint a positive picture and promote the National Green Hydrogen Mission as a great launchpad for FCEVs in India.
4
COMPARING FCEVs & BEVs
HOW DO FCEVs HOLD UP AGAINST THE COMPETITION?
FCEVs have a strong and popular competitor in BEVs, which have a tight grasp on the market. To evaluate the viability of FCEVs, we have compared them to BEVs on seven vital parameters: (1) driving range, (2) refueling time, (3) infrastructure, (4) hydrogen permeation, (5) energy efficiency, (6) environmental impact, and (7) total cost of ownership (TCO). The following analysis aims to assess the strengths and the best use cases for each technology.
Driving range
Since their origin, consumers have associated electric vehicles with “range anxiety,” which is the fear that the vehicle’s battery will not have enough range to travel the desired distance. BEVs have evolved significantly to improve their ranges but fall short of the range potential of FCEVs (see Figure 4).
The average range of an FCEV is 483 km, compared to the 322 km range offered by a BEV. The Toyota Mirai FCEV provides a range of 650 km, while only a few high-end BEVs can deliver around 565 km.
When examining the driving range, the weight-range trade-off is a crucial aspect to include. FCEVs fare much better than BEVs on this parameter. When more range is added to an FCEV, the weight increase is marginal. It is significantly less than a range increase in a BEV; here, the battery weight goes up far more steeply as the range increases. Hydrogen has a high gravimetric energy storage density, meaning the amount of energy stored per unit mass is 120 megajoules per kilogram (MJ/kg). The weight advantage of energy storage of an FCEV, compared to a BEV, translates to significant payload improvements for commercial vehicles.
Refueling time
FCEVs pull far ahead of BEVs on this metric, requiring just a few minutes to fully refuel. In contrast, BEVs typically need 30 minutes to attain an 80% charge, which requires fast charging capability on both the vehicle and infrastructure sides. Otherwise, refueling could take multiple hours. However, fast charging typically comes at a higher cost per kWh and has a negative impact on battery life.
Innovations in BEVs have the potential to reduce charging time drastically, providing 80% charging in around 15 minutes. Tesla’s super-fast chargers promise a 300 km range in 15 minutes of charge time. However, these are only available for high-end vehicles and haven’t yet been tested in the Indian market. Significant investment in India’s grid infrastructure would be required to support a fast-charging infrastructure deployment. Therefore, the economic and commercial viability of these innovations in BEVs is unknown.
Infrastructure
BEV charging infrastructure is far more developed than the refueling infrastructure for FCEVs. But both technologies face challenges to establishing the infrastructure they need to keep up with demand. Figure 5 shows the availability and type of publicly accessible EV chargers, by country, while Figure 6 shows available hydrogen fueling stations by country.
Let’s look at the key challenges FCEVs and BEVs must overcome as they grow their infrastructure:
-
High initial setup costs. Establishing the infrastructure for FCEVs requires a more considerable initial investment than BEVs. The cost of constructing a hydrogen refueling station is roughly US $2 million (spent by both Columbia and the US), which is substantially higher than an electric charging station (US $50,000) or a gas or petrol station.[19] The nascent stage of FCEV adoption has caused concern for companies that are uncertain about their return on investment.
-
Safety concerns. Hydrogen is highly combustible, hard to store and transport, and necessitates advanced technology to safely establish supply chain systems. Truck transportation of hydrogen, storage at hydrogen stations, and the corresponding dispensing units need to follow high safety standards, which might be a financial burden on initial investments and regular operating costs. In comparison, BEV charging stations are known to be relatively safe, given the higher level of maturity of charging technologies and software.
-
Real estate requirements. Hydrogen stations are difficult to build and operate due to safety concerns and the need for specialized storage tanks. They must also adhere to strict safety standards, while BEV charging stations feature multiple chargers and can be installed near offices and businesses. Ideally, BEV charging stations should be located near a grid that can simultaneously withstand the expected load from multiple chargers connections.
Hydrogen permeation
Hydrogen permeation is another consideration when dealing with FCEVs. This refers to the process of hydrogen gas passing through a solid material due to the difference in the partial pressures of hydrogen on either side of the material. It is deliberately allowed in some hydrogen fuel cells to prevent the buildup of hydrogen pressure inside the cell, which could lead to a rupture. The amount of hydrogen allowed to permeate is carefully controlled to ensure that it does not cause a significant loss of fuel. Monitoring the amount helps keep the concentration of hydrogen in the air below the flammable limit. BEVs face no comparable leakage issue.
Energy efficiency
Energy efficiency is crucial to a vehicle’s TCO, usage flexibility, and sustainability. The well-to-wheel energy efficiency of hydrogen FCEVs is significantly lower than BEVs. The FCEV has a well-to-wheel energy efficiency of 25%-35%, while the BEV has a well-to-wheel efficiency of 70%-80%. Figure 7 shows how the two compare on this measure.
With regard to storable energy, FCEVs benefit from an inherently high gravimetric energy density of 33.6 kilowatt-hours per kilogram (kWh/kg) of hydrogen. This is much lower than the energy density of electric batteries, which is approximately 250 kWh/kg, as currently used by Tesla. However, hydrogen’s low volumetric energy density in compressed gaseous and liquid form constrains the realistically storable content in a vehicle, and the weight of the hydrogen storage tanks offset some of the gravimetric benefits.
Environmental impact
The ecological efficiency of FCEVs and BEVs is determined by their energy source and production. The reputation of BEV manufacturing is marred by environmental damage caused by mining for raw materials concentrated in certain parts of the world, making battery production the most questionable part of EV production. In our Viewpoint, “Toward a Sustainable Value Chain for Electric Vehicles in India,” we delve deeper into the social and environmental risks associated with mining battery raw materials.
Today, almost all hydrogen is derived from carbon-based materials with significant CO2 emissions. Like the earlier classification, which assigns hydrogen a color based on its level of sustainability, this approach divides hydrogen into five categories based on production process. The criteria for each color could change over time and may even differ among different countries, as there is no universal naming convention.
As discussed in the “Energy efficiency” section above, well-to-wheel efficiency is substantially higher for BEVs. FCEVs use ecologically inefficient methods of hydrogen production, allowing BEVs to take the lead in environmental friendliness.
However, FCEVs are catching up with innovative solutions. According to Toyota, its FCEV Mirai cleans the air and is a negative-emission vehicle, capturing even fine particulate matter (PM) 2.5 particles. Air goes through the car’s numerous membranes, ultimately flushing cleaner air back into the atmosphere.
Green hydrogen production will make hydrogen more environmentally friendly, even though the efficiency of the process might still be lacking. FCEVs show great potential to create a positive impact on the environment.
TCO analysis
We arrived at the TCO by considering the purchase price, the running cost of fuel, and the subsequent cost of operations and maintenance (O&M) undertaken by a typical customer (see Table 1). The purchase price is the average one-time cost to buy the car. O&M costs include maintenance, parts replacement, expected depreciation, insurance, and other expenses. We have used the average prices of standard passenger cars for this comparison (see Figure 8).
As economies of scale and government subsidies kick in, we expect the TCO for both BEVs and FCEVs to decrease. Therefore, for FCEV passenger cars, we included the future forecasted costs. We also benchmarked both BEVs and FCEVs against ICE vehicles, assuming ICEs have reached full economies of scale and have a fully developed supporting infrastructure.
The following assumptions are crucial to our analysis:
-
Our TCO calculations were based on a six-to-eight-year vehicle lifespan and an assumed total distance traveled by the car of 150,000 km.
-
We used current energy prices and assumed they would remain constant.
-
Our estimate of the cost of hydrogen fuel was based on conservative industry forecasts provided by experts.
-
Currently, the Toyota Mirai is the only hydrogen FCEV available in India. However, we anticipate more models becoming available in the coming years by different manufacturers; this will drive down prices due to competition, government support, and economies of scale. Therefore, for comparison purposes, we estimated the cost of a more affordable version of FCEVs.
Despite the higher initial cost of both BEVs and FCEVs when compared to ICEs, the lower running expenses of both types of EVs will allow for the recovery of the initial cost over the vehicle’s lifespan. The high initial cost for FCEVs will also decrease, making them more attractive in terms of TCO.
FCEVs’ costs will decrease over the next decade, benefiting from economies of scale and technological innovations. Hydrogen fuel costs are expected to decline; creating the required infrastructure will support the growth of FCEVs, and OEMs will compete over the consumer base. When compared to BEVs and ICEs, these factors will support the growth of FCEVs and offer unique value propositions and cost-effectiveness.
5
ALTERNATIVE MOBILITY USES FOR HYDROGEN
HYDROGEN CAN ENABLE A SMOOTH TRANSITION TO EVs
Hydrogen has numerous on-the-ground use cases; many are already being implemented globally. Countries like Germany are primarily focused on FCEVs; however, India is also pursuing alternatives that provide opportunities for rapid uptake of hydrogen and partial use of legacy technology. Vehicle manufacturers could pick these up quickly to extend existing technology for longer, using cleaner fuel alternatives. End users will rely more on tried-and-tested technology to smooth the transition, and alternatives will be more suitable for heavier loads and other requirements. These steps will require setting up a hydrogen ecosystem that could also be used for FCEVs. There are prominent examples of these alternatives, including:
-
Hythane (H-CNG). H-CNG is a hydrogen-enriched compressed natural gas (CNG). It is cleaner, more cost-efficient, and increases fuel efficiency. With 70% more reduction in carbon monoxide emissions compared to CNG, H-CNG could be an essential solution to fulfill upcoming emission requirements. The Indian Oil Corporation Limited (IOCL), in collaboration with the Delhi Transport Department, set up a compact reformer-based plant capable of producing 4 tons of H-CNG a day.
-
Methanol (H2 derived) blending of gasoline. Methanol can be processed to make dimethyl ether (DME), which would be a cleaner substitute for diesel and would only require slight changes to existing diesel engines. According to a NITI Aayog report, blending 15% methanol with gasoline can reduce India’s import of crude oil by about 15%. This blend would also help improve air quality by reducing GHG emissions by 20%. IOCL rolled out M15 petro, a 15% blend of methanol with petrol, as part of a pilot project in Assam’s Tinsukia district.
-
Ammonia (H2 derived) for ships. Ammonia is another cleaner alternative that can be used by fuel cells and ICEs. It can be made using renewable resources and has easy storage options. Ammonia is a zero-carbon option with lower costs than its counterparts. Its high energy density and scalability have made it one of the optimum choices for marine fuel. A German multinational company, MAN Energy Solutions, has developed a timeline to deliver commercially viable ammonia-burning engines by 2024. It aims for economically feasible and scalable solutions that could be rapidly deployed.
-
H2 ICE. This entails substituting petrol, diesel, or CNG with hydrogen in a vehicle with an ICE. H2 ICE will require familiar parts from vehicle manufacturers and provide end users with reliable and trusted technology. This change will translate to zero emission of carbon-based pollutants. However, since hydrogen is burned at high temperatures with ambient air, nitrogen oxide emissions will generate pollution. Still, H2 ICE would have zero-carbon tailpipe emissions and be able to share hydrogen production and distribution ecosystem infrastructure with both ICEs and FCEVs. In India, Tata Motors aims to use H2 ICE technology for its buses and trucks to leverage ICE’s intellectual property and infrastructure.
6
WHAT THE FUTURE HOLDS FOR INDIA
GROWTH IN HYDROGEN MOBILITY CAN ACHIEVE ITS SUSTAINABILITY GOALS
Dependence on imports is a critical constraint for existing BEV models. FCEVs provide the opportunity to decrease this reliance, which supports the Indian government’s Atmanirbhar Initiative. Steps taken in this direction will work toward fulfilling an essential element of India Vision 2030, which is pivotal to the transportation ecosystem: a pollution-free India.
The entrance of FCEVs in a fast-evolving mobility space may create uncertainties in OEM strategies and in customers’ minds. Therefore, the government should play a vital role in facilitating a smooth transition within the car sector to allow these various technologies to coexist. One possible strategy is to introduce FCEVs for buses, trucks, and other heavy-duty vehicles, while BEVs remain exclusive to the passenger car category, where they already dominate the EV race and would continue to thrive. This approach could be viable, as establishing infrastructure for FCEVs would take less time and investment because fewer stations and establishments would be required than for passenger vehicles. Hydrogen’s high gravimetric energy density is more suitable for long-distance, heavyweight, and auxiliary load mobility and transport, as discussed earlier in the report. Our TCO analysis also supports this hypothesis. Based on the above analysis and our TCO calculations, we anticipate a production timeline of different vehicle types across use cases as reflected in Figure 9.
As FCEVs carve out their mobility and transport space niche, the government needs an effective strategy to build a sustainable ecosystem around hydrogen-powered vehicles and ensure a smooth transition for the auto industry. From a policy-making perspective, the following actions can help drive the growth of FCEVs in the Indian market:
-
The government can provide subsidiaries and incentives for building the necessary infrastructure (from converting green energy to hydrogen, to building hydrogen fuel stations) to support the growth of hydrogen vehicles.
-
Private players, companies, and research institutions should be encouraged to invest in R&D for hydrogen in mobility and identify ways to improve its cost-effectiveness.
-
The government can encourage near-term market development by identifying industrial clusters and enacting viability gap funding and mandates.
FCEVs can save OEMs from the sudden downfall of existing technology and a demand crisis, especially for commercial vehicles where battery technology is still not advanced and sustainable targets are not getting any closer. Therefore, the government’s proactive support for the growth of technology could prove crucial in defining the future of India’s mobility space.
Notes
[1] “Global Electric Car Sales Have Continued Their Strong Growth in 2022 After Breaking Records Last Year.” Press release, International Energy Agency (IEA), 23 May 2022.
[2] Baharudin, Luqmanulhakim, and Matthew Watson. “Hydrogen Applications and Research Activities in Its Production Routes Through Catalytic Hydrocarbon Conversion.” Reviews in Chemical Engineering, Vol. 34, No. 1, March 2017.
[3] “2023 Global Threat Report.” CrowdStrike, 2023.
[4] T., Ashish, and Sonia M. “Hydrogen Fuel Cell Vehicle Market by Vehicle Type (Sedan, SUV, Others), by Technology (Proton Exchange Membrane Fuel Cell, Phosphoric Acid Fuel Cell), by Range (0-250 Miles, 251-500 Miles, Above 500 Miles): Global Opportunity Analysis and Industry Forecast, 2021–2031.” Allied Market Research, December 2022.
[5] McDonald, Jeffrey. “Fuel Cell EVs Set to Top 13 Million by 2030 as Hydrogen Scales Up: Hydrogen Council.” S&P Global Commodity Insights, 24 January 2020.
[6] Creamer, Martin. “China Becoming Fuel Cell Electric Vehicle Leader, World Platinum Research Indicates.” Mining Weekly, 20 October 2022.
[7] “Analysis of Fuel Cell Vehicle Market in North America — Growth, Trends, and Forecasts (2023-2028).” Mordor Intelligence, accessed May 2023.
[8] McDonald, Jeffrey. “Fuel Cell EVs Set to Top 13 Million by 2030 as Hydrogen Scales Up: Hydrogen Council.” S&P Global Commodity Insights, 24 January 2020.
[9] Nakano, Jane. “Japan’s Hydrogen Industrial Strategy.” Center for Strategic & International Studies, 21 October 2021.
[10] “Japan Aims to Boost Hydrogen Supply to 12 Million T by 2040.” Reuters, 4 April 2023.
[11] “Germany Plans to Invest EUR 3.6 Billion in Green Hydrogen.” Power Info Today, accessed May 2023.
[12] Menon, Anoop. “Morocco’s First Green Hydrogen Project to Start Production in 2025.” ZAWYA, 24 May 2021.
[13] Martin, Nik. “Germany Invests €9 Billion in Hydrogen.” Deutsche Welle, 10 June 2020.
[14] “H2 Mobility Gets $121 Million from Investors to Build Hydrogen Network.” Reuters, 29 March 2022.
[15] Dutta, Sanjay. “Rs 19,744 Crore Incentive Makes India Global Green Hydrogen Hotspot.” Times of India, 5 January 2023.
[16] Ministry of New and Renewable Energy. “Cabinet Approves National Green Hydrogen Mission.” Press Information Bureau, Government of India, 4 January 2023.
[17] “Cabinet Approves National Green Hydrogen Mission.” Ministry of New and Renewable Energy, Government of India, 4 January 2023.
[18] “India’s Energy Transition Towards a Green Hydrogen Economy.” FTI Consulting, 9 June 2021.
[19] Melaina, M., and M. Penev. “Hydrogen Station Cost Estimates: Comparing Hydrogen Station Cost Calculator Results with Other Recent Estimates.” National Renewable Energy Laboratory, September 2013.
DOWNLOAD THE FULL REPORT